DeoxycytidineCAS# 951-77-9 |
Quality Control & MSDS
Number of papers citing our products
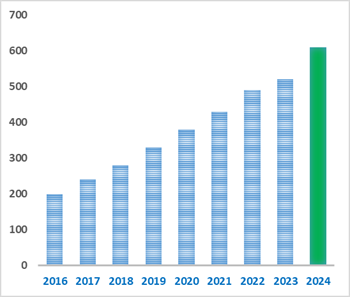
Chemical structure
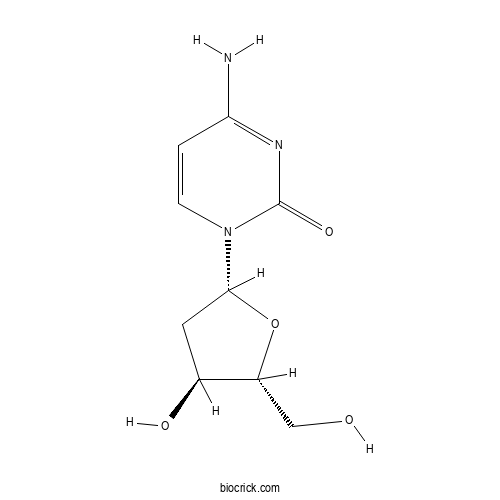
3D structure
Cas No. | 951-77-9 | SDF | Download SDF |
PubChem ID | 13711.0 | Appearance | Powder |
Formula | C9H13N3O4 | M.Wt | 227.22 |
Type of Compound | N/A | Storage | Desiccate at -20°C |
Solubility | Soluble in Chloroform,Dichloromethane,Ethyl Acetate,DMSO,Acetone,etc. | ||
Chemical Name | 4-amino-1-[(2R,4S,5R)-4-hydroxy-5-(hydroxymethyl)oxolan-2-yl]pyrimidin-2-one | ||
SMILES | C1C(C(OC1N2C=CC(=NC2=O)N)CO)O | ||
Standard InChIKey | CKTSBUTUHBMZGZ-SHYZEUOFSA-N | ||
Standard InChI | InChI=1S/C9H13N3O4/c10-7-1-2-12(9(15)11-7)8-3-5(14)6(4-13)16-8/h1-2,5-6,8,13-14H,3-4H2,(H2,10,11,15)/t5-,6+,8+/m0/s1 | ||
General tips | For obtaining a higher solubility , please warm the tube at 37 ℃ and shake it in the ultrasonic bath for a while.Stock solution can be stored below -20℃ for several months. We recommend that you prepare and use the solution on the same day. However, if the test schedule requires, the stock solutions can be prepared in advance, and the stock solution must be sealed and stored below -20℃. In general, the stock solution can be kept for several months. Before use, we recommend that you leave the vial at room temperature for at least an hour before opening it. |
||
About Packaging | 1. The packaging of the product may be reversed during transportation, cause the high purity compounds to adhere to the neck or cap of the vial.Take the vail out of its packaging and shake gently until the compounds fall to the bottom of the vial. 2. For liquid products, please centrifuge at 500xg to gather the liquid to the bottom of the vial. 3. Try to avoid loss or contamination during the experiment. |
||
Shipping Condition | Packaging according to customer requirements(5mg, 10mg, 20mg and more). Ship via FedEx, DHL, UPS, EMS or other couriers with RT, or blue ice upon request. |

Deoxycytidine Dilution Calculator

Deoxycytidine Molarity Calculator
1 mg | 5 mg | 10 mg | 20 mg | 25 mg | |
1 mM | 4.401 mL | 22.0051 mL | 44.0102 mL | 88.0204 mL | 110.0255 mL |
5 mM | 0.8802 mL | 4.401 mL | 8.802 mL | 17.6041 mL | 22.0051 mL |
10 mM | 0.4401 mL | 2.2005 mL | 4.401 mL | 8.802 mL | 11.0026 mL |
50 mM | 0.088 mL | 0.4401 mL | 0.8802 mL | 1.7604 mL | 2.2005 mL |
100 mM | 0.044 mL | 0.2201 mL | 0.4401 mL | 0.8802 mL | 1.1003 mL |
* Note: If you are in the process of experiment, it's necessary to make the dilution ratios of the samples. The dilution data above is only for reference. Normally, it's can get a better solubility within lower of Concentrations. |
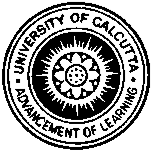
Calcutta University
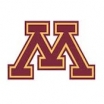
University of Minnesota
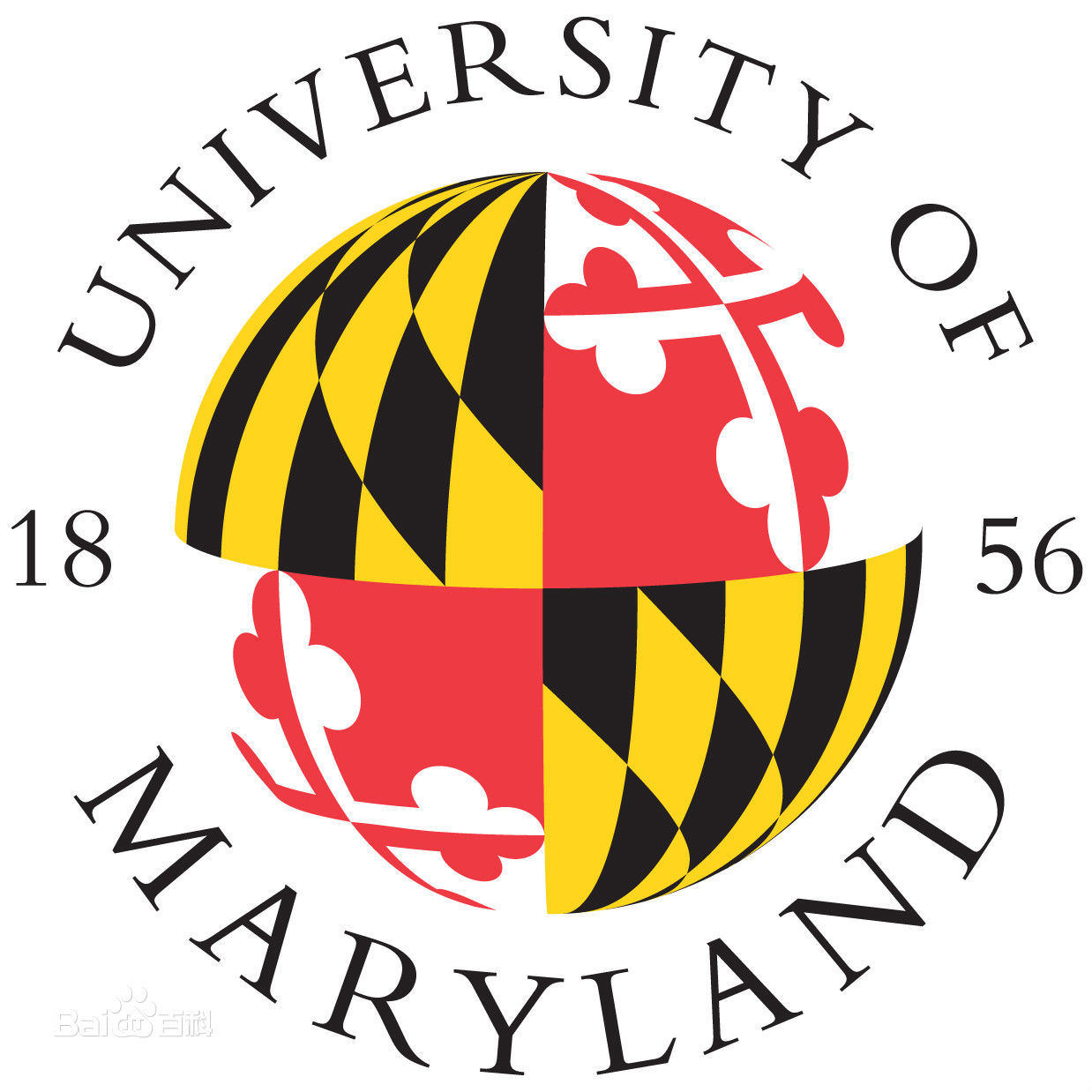
University of Maryland School of Medicine
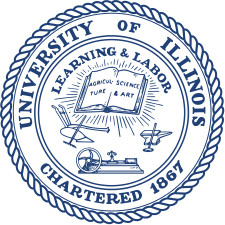
University of Illinois at Chicago
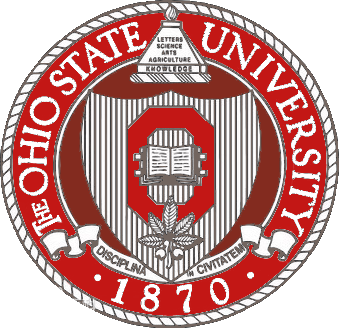
The Ohio State University
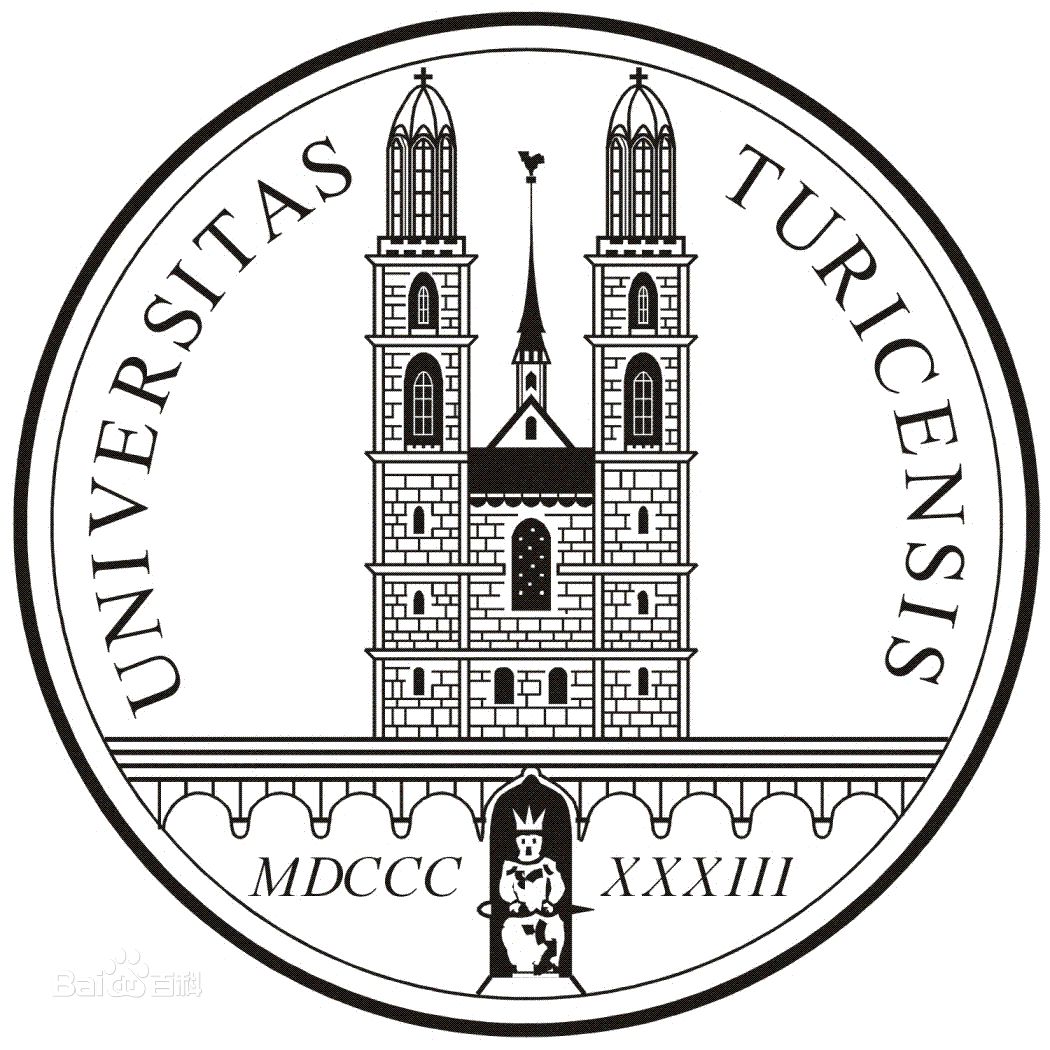
University of Zurich
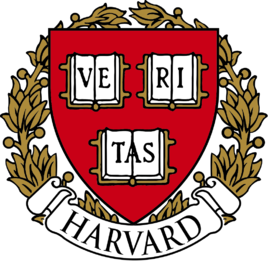
Harvard University
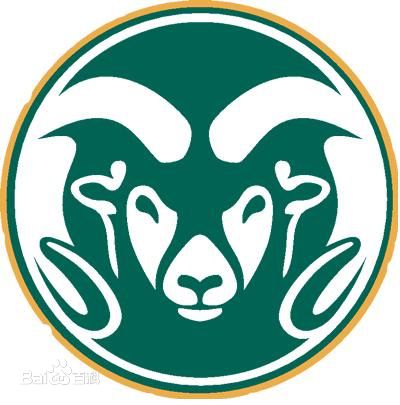
Colorado State University
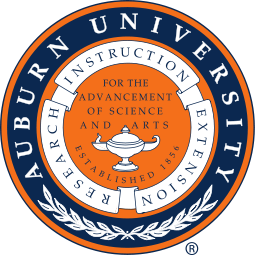
Auburn University
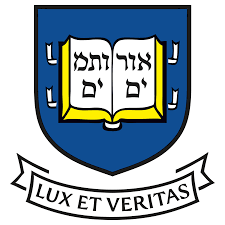
Yale University
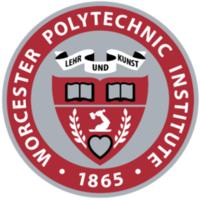
Worcester Polytechnic Institute
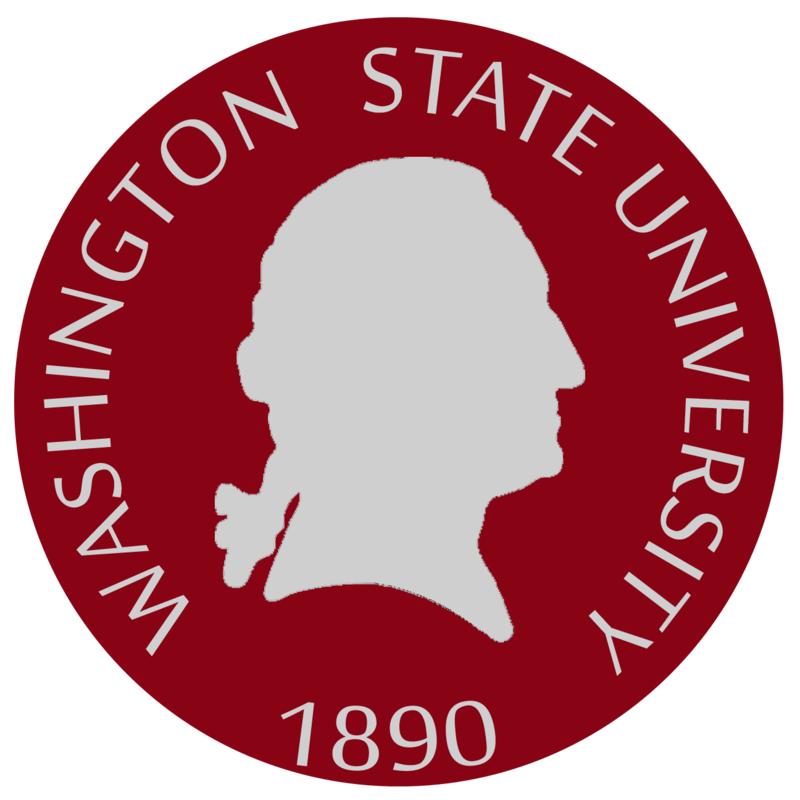
Washington State University
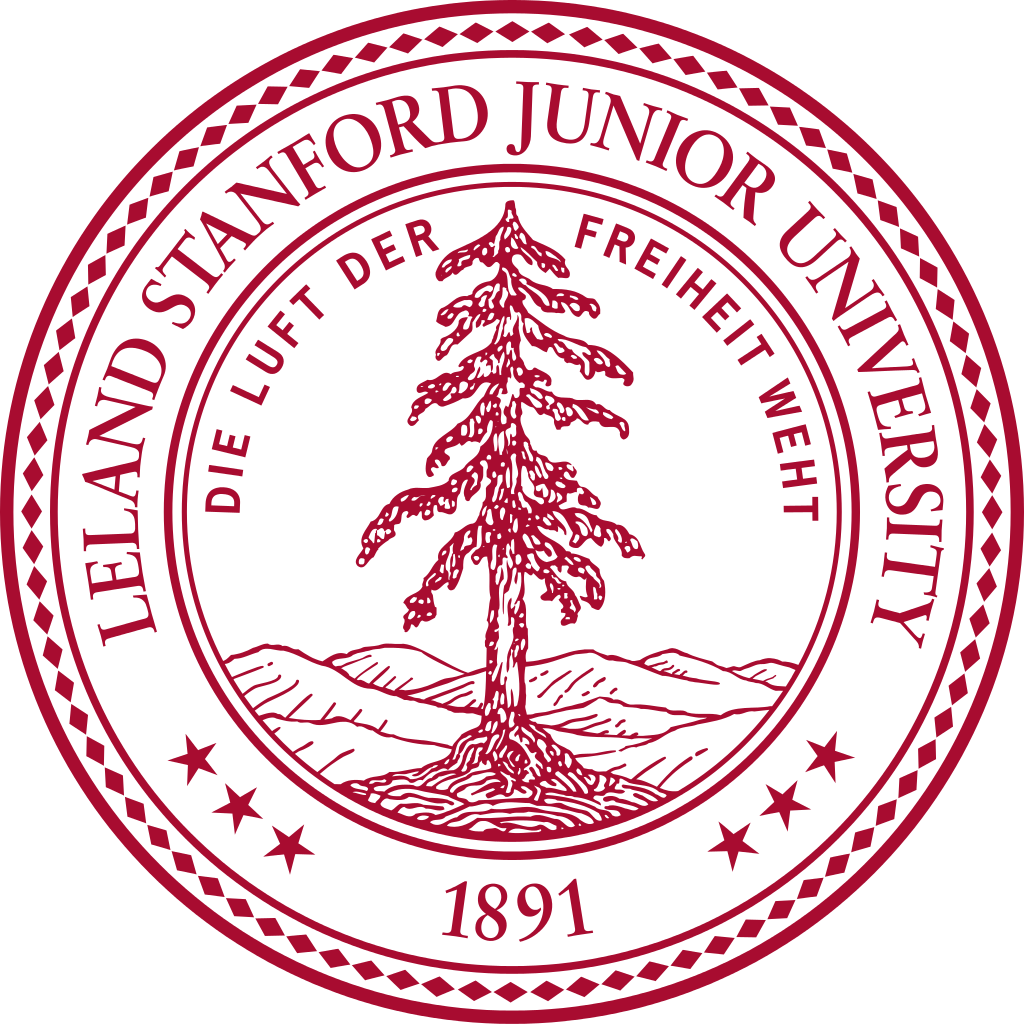
Stanford University
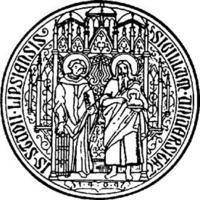
University of Leipzig
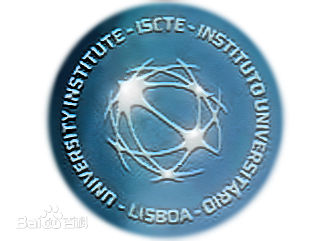
Universidade da Beira Interior
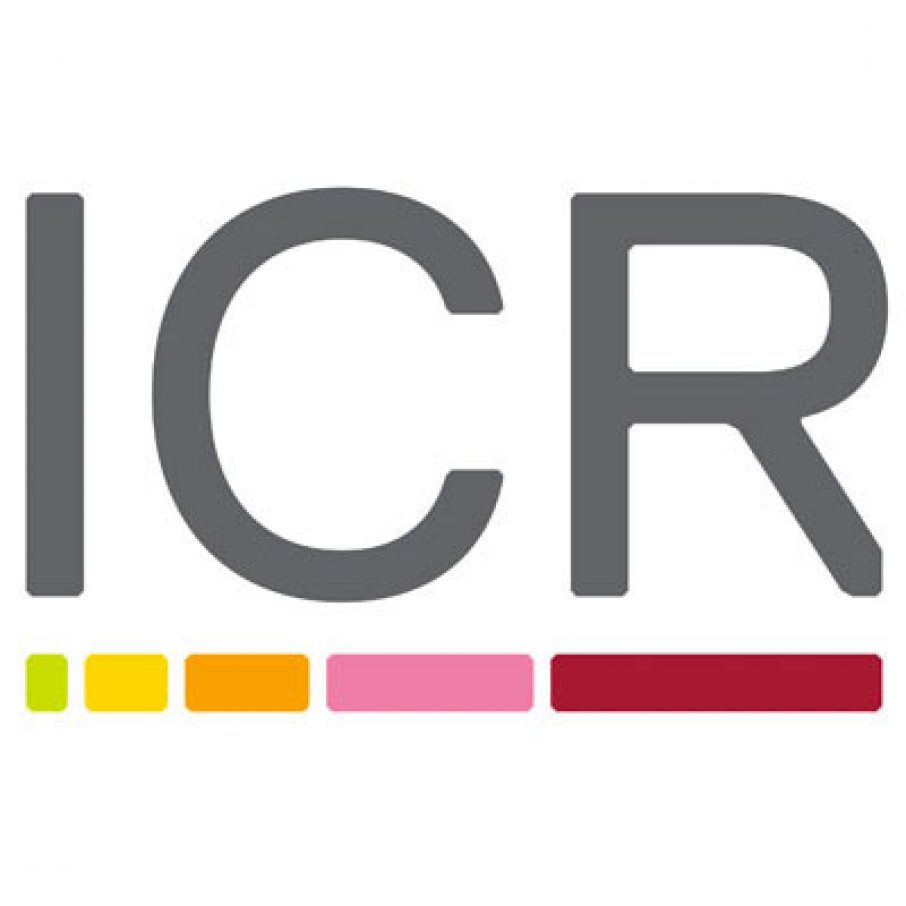
The Institute of Cancer Research
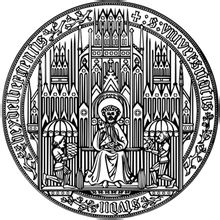
Heidelberg University
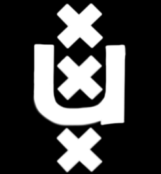
University of Amsterdam
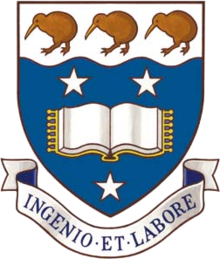
University of Auckland
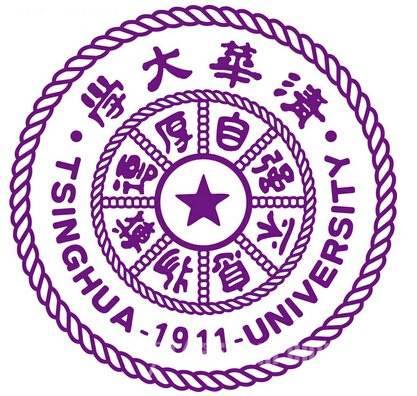
TsingHua University
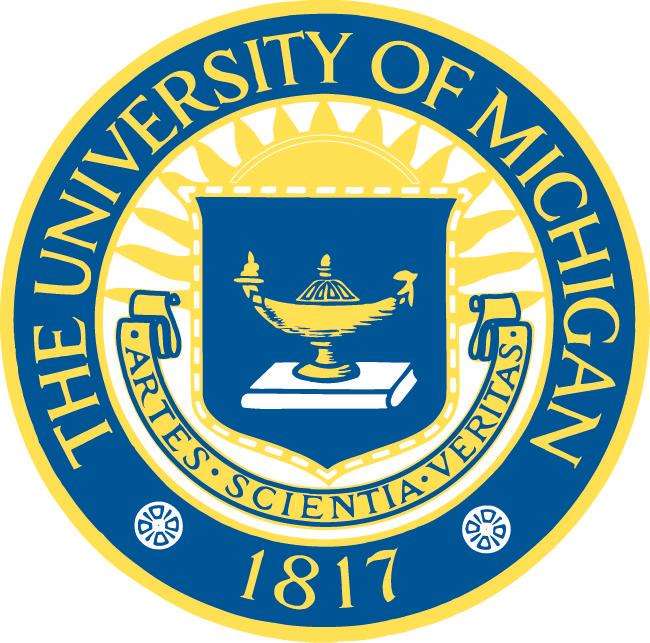
The University of Michigan
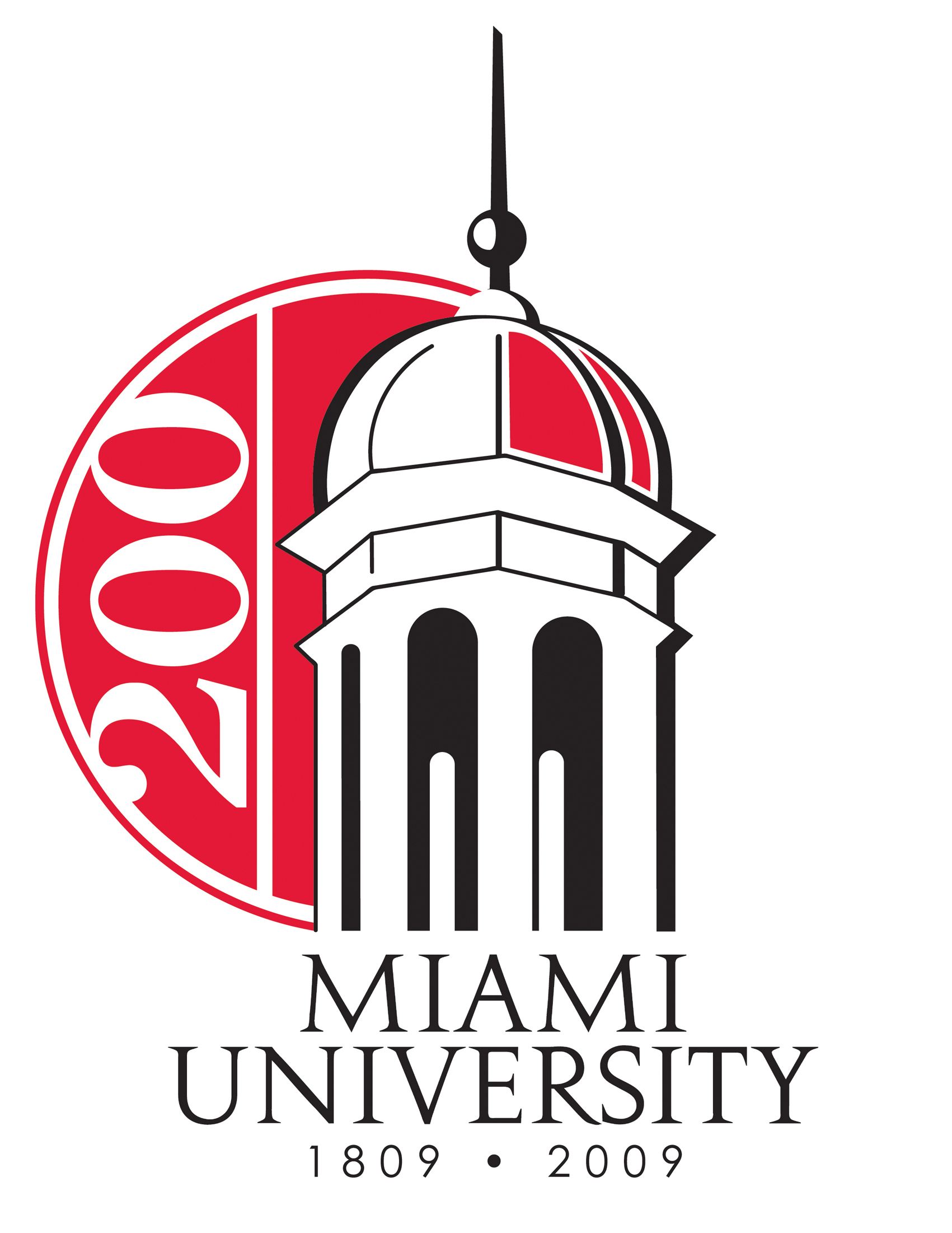
Miami University
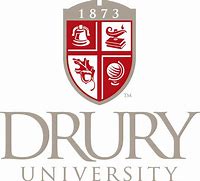
DRURY University
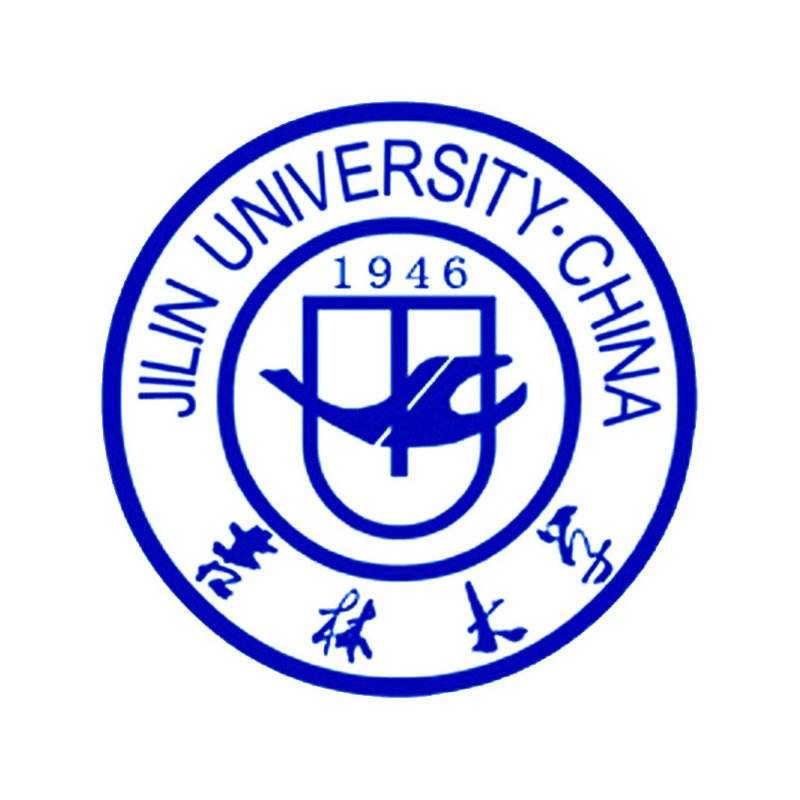
Jilin University
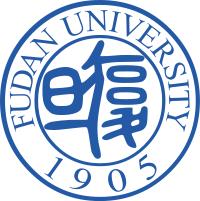
Fudan University
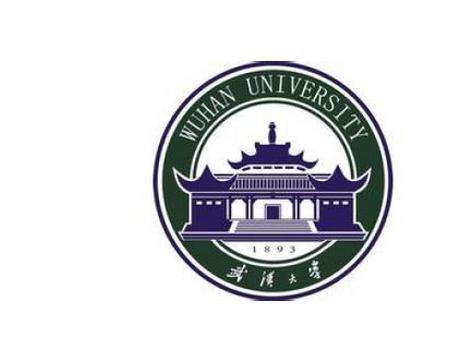
Wuhan University
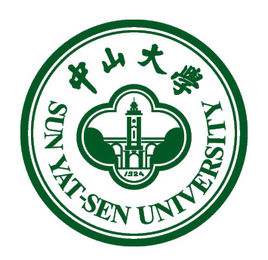
Sun Yat-sen University
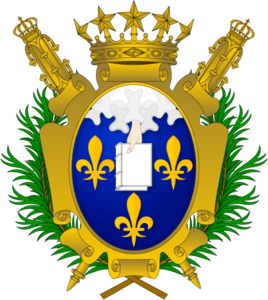
Universite de Paris
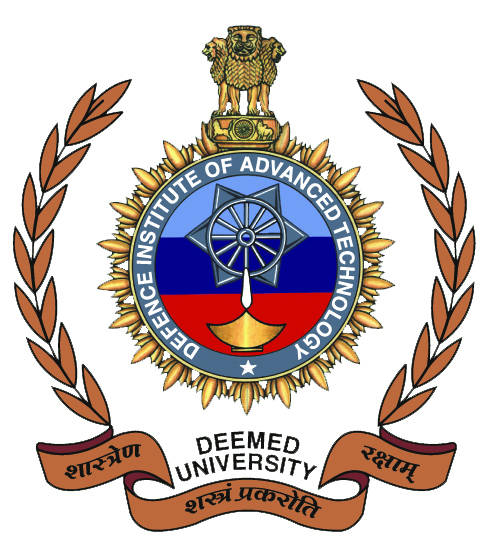
Deemed University
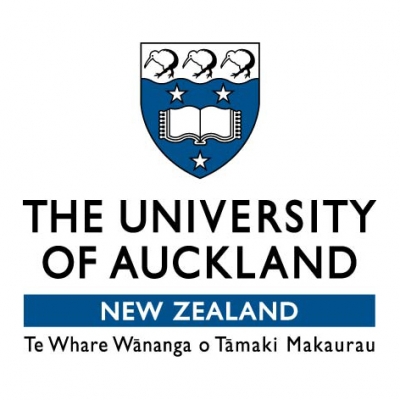
Auckland University
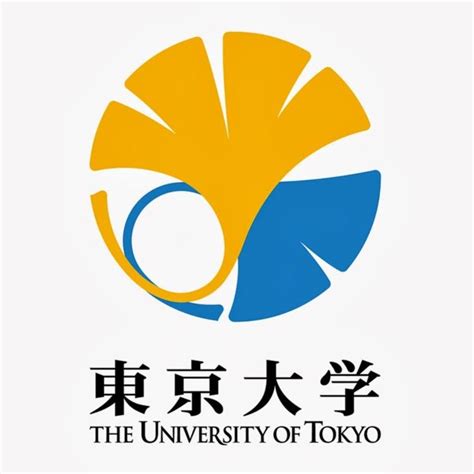
The University of Tokyo
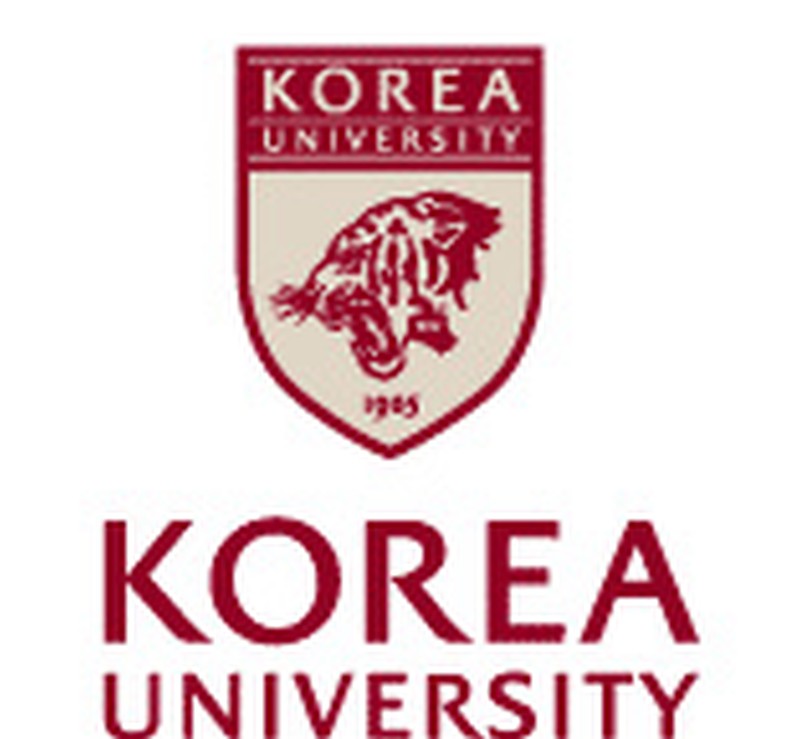
Korea University
- Kissoone A
Catalog No.:BCX1189
CAS No.:903559-01-3
- Glycyrrhizic acid methyl ester
Catalog No.:BCX1188
CAS No.:104191-95-9
- Rubropunctamine
Catalog No.:BCX1187
CAS No.:514-66-9
- Neoisoliquiritigenin
Catalog No.:BCX1186
CAS No.:7014-39-3
- 6-Acetyl-7-hydroxy-2,3-dimethylchromone
Catalog No.:BCX1185
CAS No.:225518-70-7
- Heterophyllin J
Catalog No.:BCX1184
CAS No.:660397-15-9
- 5-Methoxyafrormosin 7-O-glucoside
Catalog No.:BCX1183
CAS No.:64656-92-4
- Acetylarenobufagin
Catalog No.:BCX1182
CAS No.:184673-79-8
- N-Acetyl-D-lactosamine
Catalog No.:BCX1181
CAS No.:32181-59-2
- GDP-L-Fuc.2Na
Catalog No.:BCX1180
CAS No.:15839-70-0
- 11-Oxo-ganoderic acid DM
Catalog No.:BCX1179
CAS No.:1408244-15-4
- 2-Hydroxypinocembrin
Catalog No.:BCX1178
CAS No.:40489-17-6
- Daucosterin acetate
Catalog No.:BCX1191
CAS No.:4282-00-2
- (-)-3-Demethylcolchicine
Catalog No.:BCX1192
CAS No.:7336-33-6
- Ludaconitine
Catalog No.:BCX1193
CAS No.:82144-72-7
- Syringopicroside
Catalog No.:BCX1194
CAS No.:29118-80-7
- Taccalonolide C
Catalog No.:BCX1195
CAS No.:117803-96-0
- Complanatuside 6"-malonate
Catalog No.:BCX1196
CAS No.:2089462-18-8
- Resinacein C
Catalog No.:BCX1197
CAS No.:1309931-92-7
- Markogenin
Catalog No.:BCX1198
CAS No.:562-35-6
- 4α-Phorbol
Catalog No.:BCX1199
CAS No.:26241-63-4
- Alltride
Catalog No.:BCX1200
CAS No.:2050-87-5
- 21-keto-gypenoside A
Catalog No.:BCX1201
CAS No.:1392136-41-2
- Tigogenin lactone
Catalog No.:BCX1202
CAS No.:514-33-0
CRISPR/dCas9-Tet1-Mediated DNA Methylation Editing.[Pubmed:38686348]
Bio Protoc. 2024 Apr 20;14(8):e4976.
DNA methylation is a key epigenetic mechanism underlying many biological processes, and its aberrant regulation has been tightly associated with various human diseases. Precise manipulation of DNA methylation holds the promise to advance our understanding of this critical mechanism and to develop novel therapeutic methods. Previously, we were only able to alter genome-wide DNA methylation by treating with small molecules (e.g., 5-Aza-2-Deoxycytidine) or perturbing relevant genes (e.g., DNA methyltransferase) targetlessly, which makes it challenging to investigate the functional significance of this epigenetic mark at specific genomic loci. By fusing the catalytic domain of a key enzyme in the DNA demethylation process (Ten-eleven translocation dioxygenases 1, Tet1) with a reprogrammable sequence-specific DNA-targeting molecular protein, dCas9, we developed a DNA methylation editing tool (dCas9-Tet1) to demethylate specific genomic loci in a targeted manner. This dCas9-Tet1 system allows us to study the role of DNA methylation at almost any given loci with only the replacement of a single-guide RNA. Here, we describe a protocol that enables modular and scalable manipulation of DNA methylation at specific genomic loci in various cell cultures with high efficiency and specificity using the dCas9-Tet1 system. Key features * Precisely editing the DNA methylation of specific genomic loci in a targeted manner. * Fine-tuning gene expression without changing DNA sequence. * Applicable to many types of cell cultures and with the potential for ex vitro and in vivo applications.
Butterfly Effect in Cytarabine: Combined NMR-NQR Experiment, Solid-State Computational Modeling, Quantitative Structure-Property Relationships and Molecular Docking Study.[Pubmed:38675407]
Pharmaceuticals (Basel). 2024 Mar 29;17(4):445.
Cytarabine (Ara-C) is a synthetic isomer of cytidine that differs from cytidine and Deoxycytidine only in the sugar. The use of arabinose instead of deoxyribose hinders the formation of phosphodiester linkages between pentoses, preventing the DNA chain from elongation and interrupting the DNA synthesis. The minor structural alteration (the inversion of hydroxyl at the 2' positions of the sugar) leads to change of the biological activity from anti-depressant and DNA/RNA block builder to powerful anti-cancer. Our study aimed to determine the molecular nature of this phenomenon. Three (1)H-(14)N NMR-NQR experimental techniques, followed by solid-state computational modelling (Quantum Theory of Atoms in Molecules, Reduced Density Gradient and 3D Hirshfeld surfaces), Quantitative Structure-Property Relationships, Spackman's Hirshfeld surfaces and Molecular Docking were used. Multifaceted analysis-combining experiments, computational modeling and molecular docking-provides deep insight into three-dimensional packing at the atomic and molecular levels, but is challenging. A spectrum with nine lines indicating the existence of three chemically inequivalent nitrogen sites in the Ara-C molecule was recorded, and the lines were assigned to them. The influence of the structural alteration on the NQR parameters was modeled in the solid (GGA/RPBE). For the comprehensive description of the nature of these interactions several factors were considered, including relative reactivity and the involvement of heavy atoms in various non-covalent interactions. The binding modes in the solid state and complex with dCK were investigated using the novel approaches: radial plots, heatmaps and root-mean-square deviation of the binding mode. We identified the intramolecular OH...O hydrogen bond as the key factor responsible for forcing the glycone conformation and strengthening NH...O bonds with Gln97, Asp133 and Ara128, and stacking with Phe137. The titular butterfly effect is associated with both the inversion and the presence of this intramolecular hydrogen bond. Our study elucidates the differences in the binding modes of Ara-C and cytidine, which should guide the design of more potent anti-cancer and anti-viral analogues.
Protein engineering a PhotoRNR chimera based on a unifying evolutionary apparatus among the natural classes of ribonucleotide reductases.[Pubmed:38648489]
Proc Natl Acad Sci U S A. 2024 Apr 30;121(18):e2317291121.
Ribonucleotide reductases (RNRs) are essential enzymes that catalyze the de novo transformation of nucleoside 5'-di(tri)phosphates [ND(T)Ps, where N is A, U, C, or G] to their corresponding deoxynucleotides. Despite the diversity of factors required for function and the low sequence conservation across RNRs, a unifying apparatus consolidating RNR activity is explored. We combine aspects of the protein subunit simplicity of class II RNR with a modified version of Escherichia coli class la photoRNRs that initiate radical chemistry with light to engineer a mimic of a class II enzyme. The design of this RNR involves fusing a truncated form of the active site containing alpha subunit with the functionally important C-terminal tail of the radical-generating beta subunit to render a chimeric RNR. Inspired by a recent cryo-EM structure, a [Re] photooxidant is located adjacent to Y(356)[beta], which is an essential component of the radical transport pathway in class I RNRs. Combination of this RNR photochimera with cytidine diphosphate (CDP), adenosine triphosphate (ATP), and light resulted in the generation of Y(356)* along with production of Deoxycytidine diphosphate (dCDP) and cytosine. The photoproducts reflect an active site chemistry consistent with both the consensus mechanism of RNR and chemistry observed when RNR is inactivated by mechanism-based inhibitors in the active site. The enzymatic activity of the RNR photochimera in the absence of any beta metallocofactor highlights the adaptability of the 10-stranded alphabeta barrel finger loop to support deoxynucleotide formation and accommodate the design of engineered RNRs.