12-Aminolauric acidCAS# 693-57-2 |
Quality Control & MSDS
Number of papers citing our products
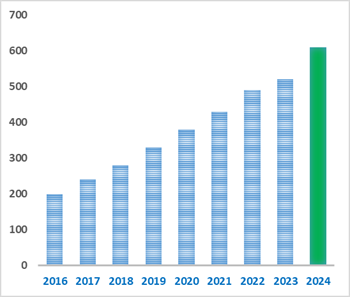
Chemical structure

3D structure
Cas No. | 693-57-2 | SDF | Download SDF |
PubChem ID | 69661 | Appearance | Powder |
Formula | C12H25NO2 | M.Wt | 215.3 |
Type of Compound | N/A | Storage | Desiccate at -20°C |
Solubility | Soluble in Chloroform,Dichloromethane,Ethyl Acetate,DMSO,Acetone,etc. | ||
Chemical Name | 12-aminododecanoic acid | ||
SMILES | C(CCCCCC(=O)O)CCCCCN | ||
Standard InChIKey | PBLZLIFKVPJDCO-UHFFFAOYSA-N | ||
Standard InChI | InChI=1S/C12H25NO2/c13-11-9-7-5-3-1-2-4-6-8-10-12(14)15/h1-11,13H2,(H,14,15) | ||
General tips | For obtaining a higher solubility , please warm the tube at 37 ℃ and shake it in the ultrasonic bath for a while.Stock solution can be stored below -20℃ for several months. We recommend that you prepare and use the solution on the same day. However, if the test schedule requires, the stock solutions can be prepared in advance, and the stock solution must be sealed and stored below -20℃. In general, the stock solution can be kept for several months. Before use, we recommend that you leave the vial at room temperature for at least an hour before opening it. |
||
About Packaging | 1. The packaging of the product may be reversed during transportation, cause the high purity compounds to adhere to the neck or cap of the vial.Take the vail out of its packaging and shake gently until the compounds fall to the bottom of the vial. 2. For liquid products, please centrifuge at 500xg to gather the liquid to the bottom of the vial. 3. Try to avoid loss or contamination during the experiment. |
||
Shipping Condition | Packaging according to customer requirements(5mg, 10mg, 20mg and more). Ship via FedEx, DHL, UPS, EMS or other couriers with RT, or blue ice upon request. |

12-Aminolauric acid Dilution Calculator

12-Aminolauric acid Molarity Calculator
1 mg | 5 mg | 10 mg | 20 mg | 25 mg | |
1 mM | 4.6447 mL | 23.2234 mL | 46.4468 mL | 92.8936 mL | 116.117 mL |
5 mM | 0.9289 mL | 4.6447 mL | 9.2894 mL | 18.5787 mL | 23.2234 mL |
10 mM | 0.4645 mL | 2.3223 mL | 4.6447 mL | 9.2894 mL | 11.6117 mL |
50 mM | 0.0929 mL | 0.4645 mL | 0.9289 mL | 1.8579 mL | 2.3223 mL |
100 mM | 0.0464 mL | 0.2322 mL | 0.4645 mL | 0.9289 mL | 1.1612 mL |
* Note: If you are in the process of experiment, it's necessary to make the dilution ratios of the samples. The dilution data above is only for reference. Normally, it's can get a better solubility within lower of Concentrations. |
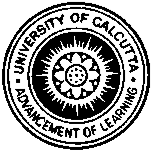
Calcutta University
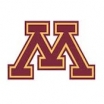
University of Minnesota
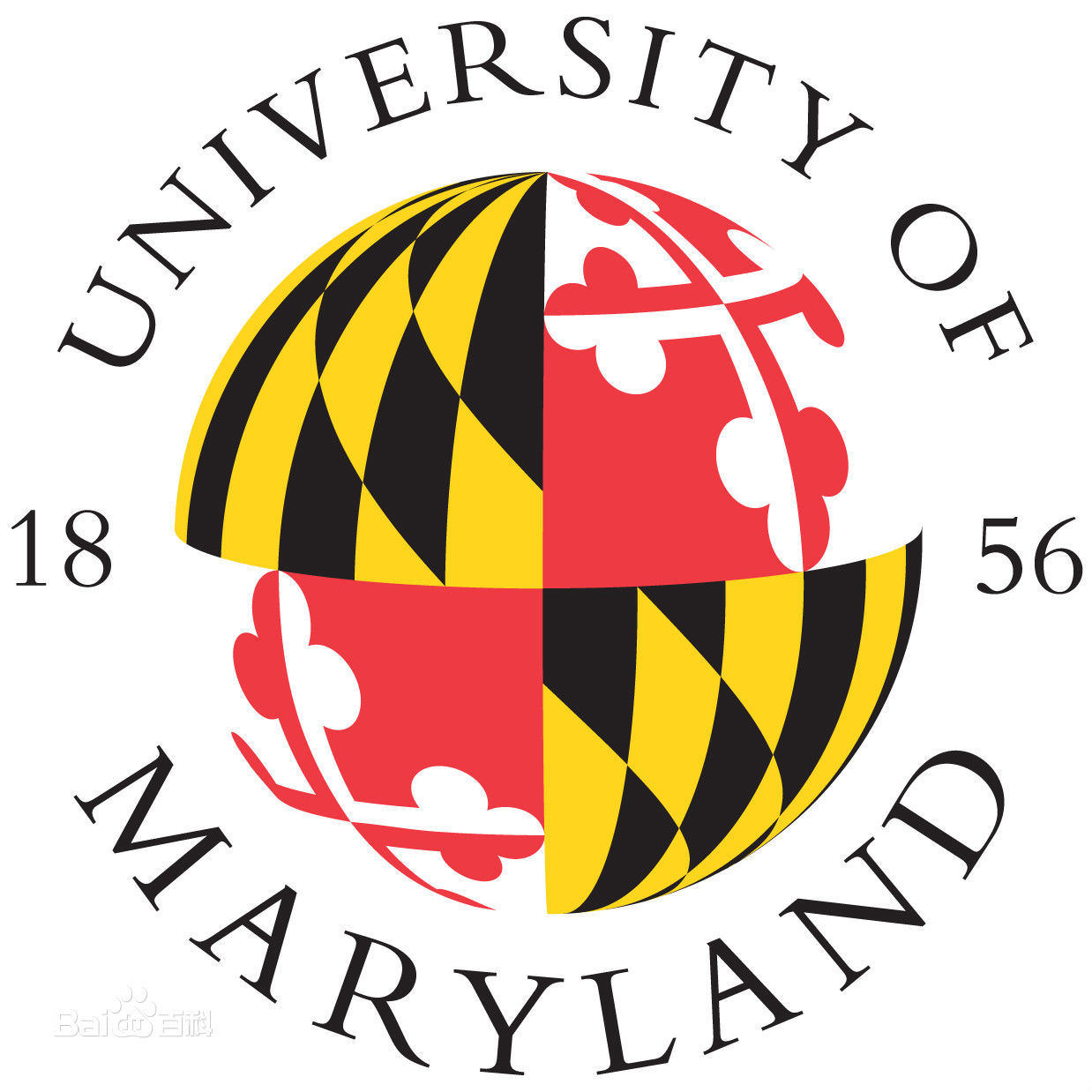
University of Maryland School of Medicine
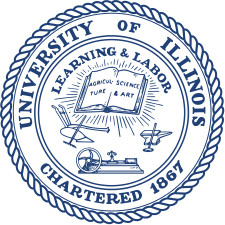
University of Illinois at Chicago
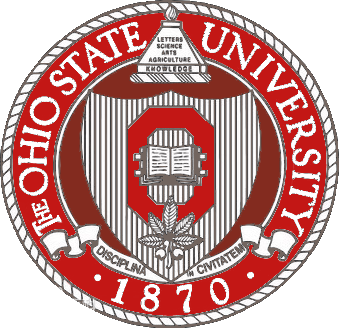
The Ohio State University
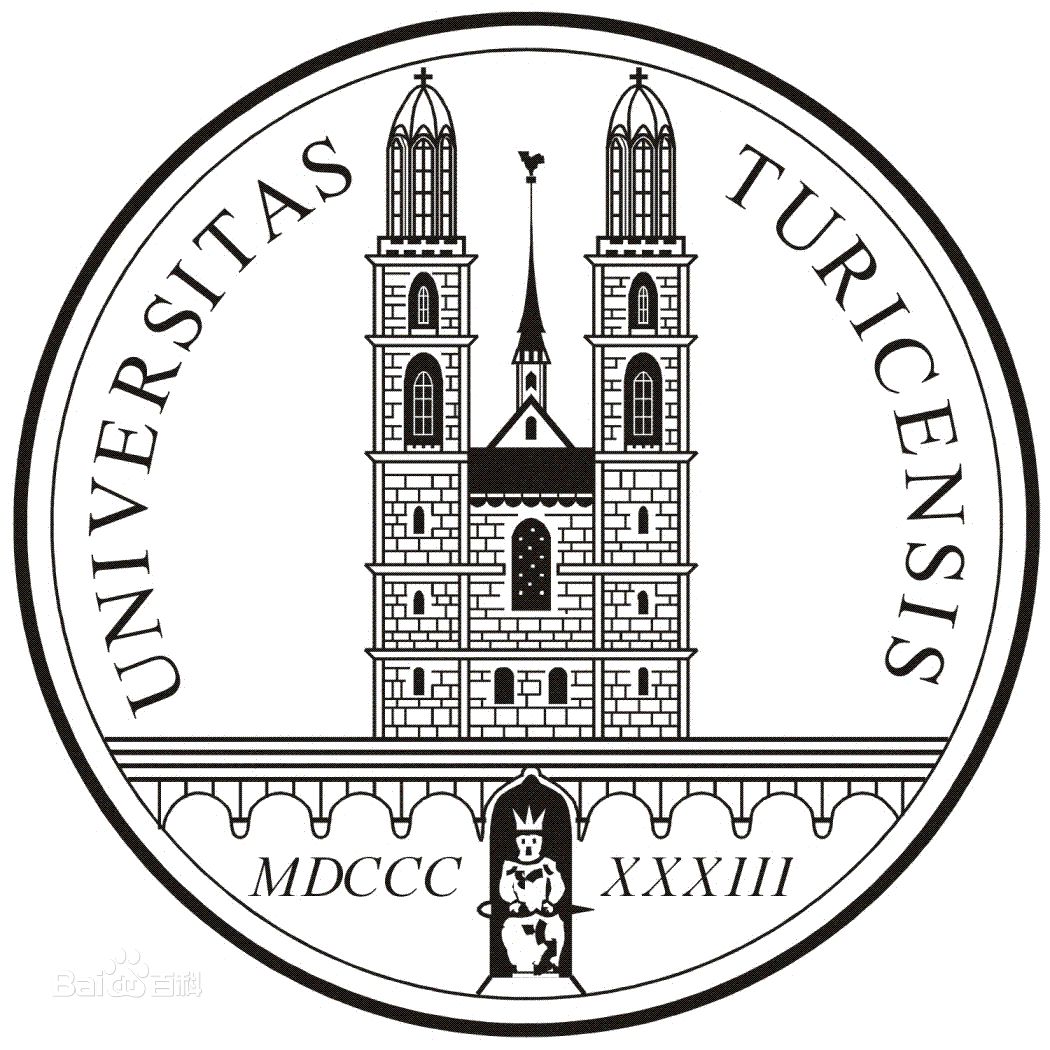
University of Zurich
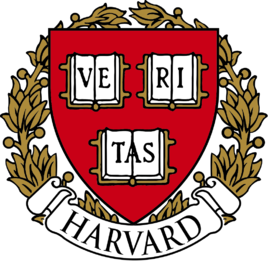
Harvard University
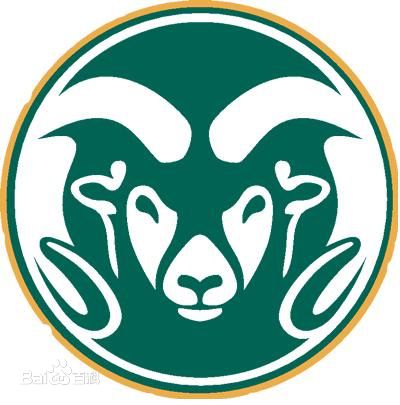
Colorado State University
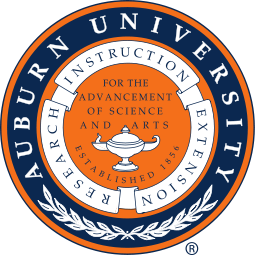
Auburn University
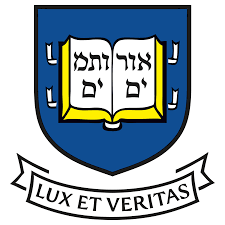
Yale University
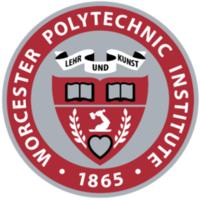
Worcester Polytechnic Institute
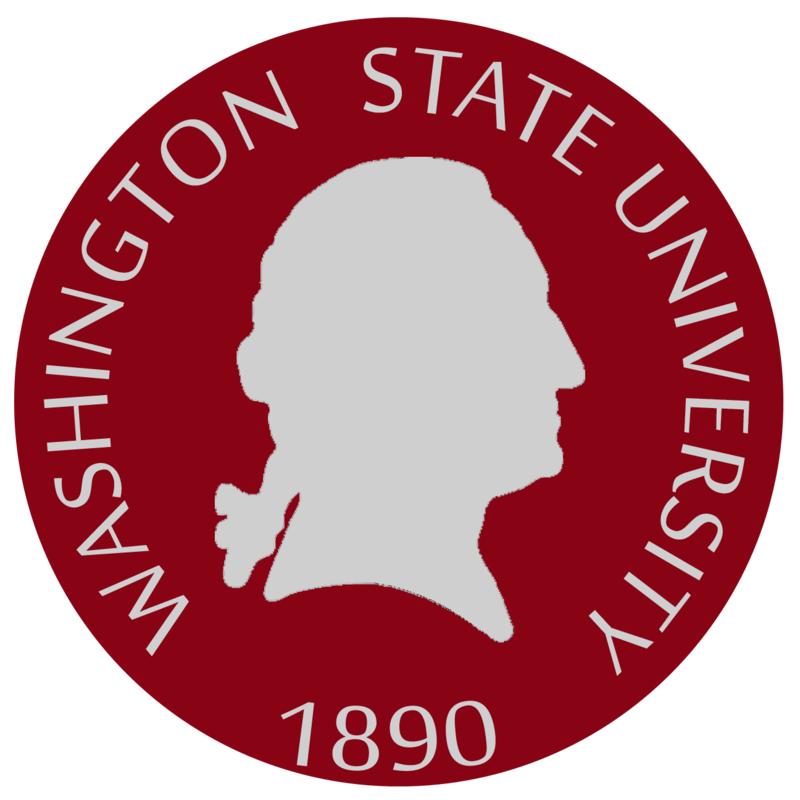
Washington State University
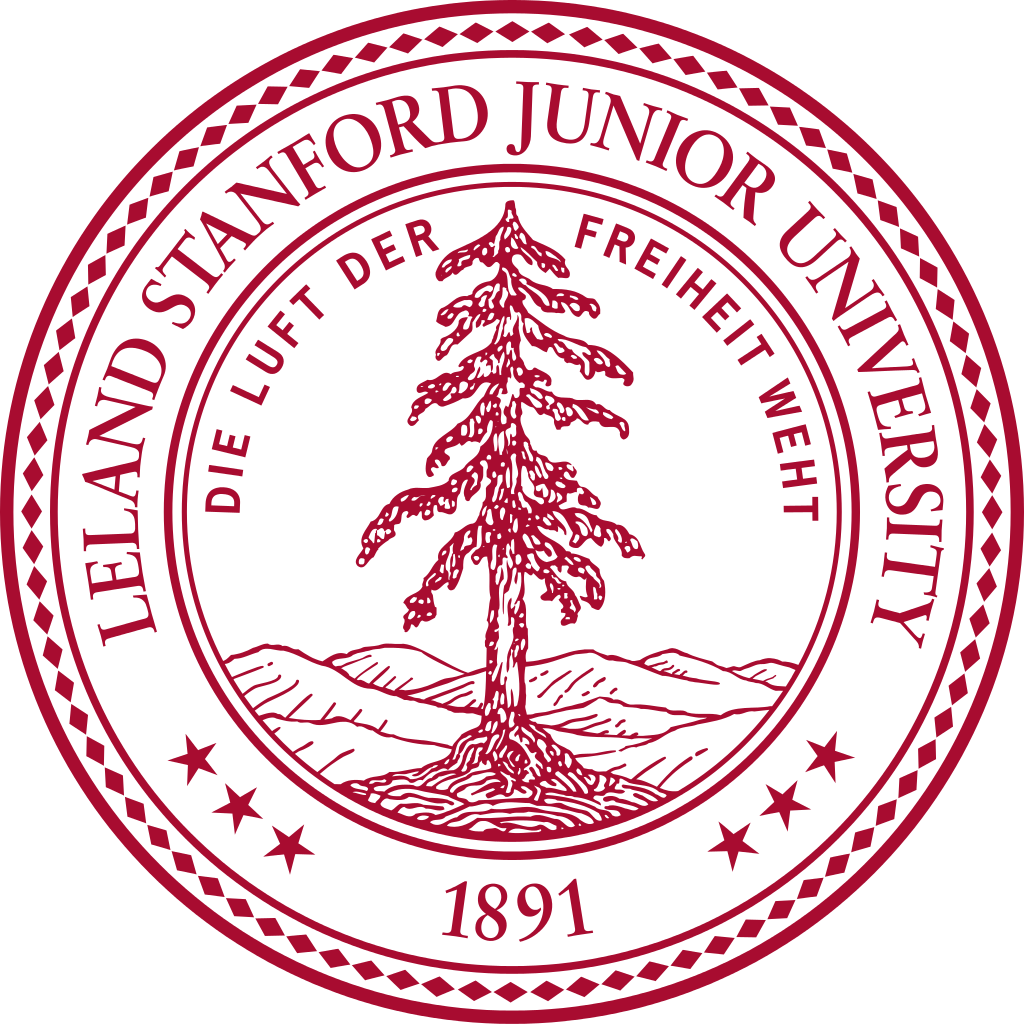
Stanford University
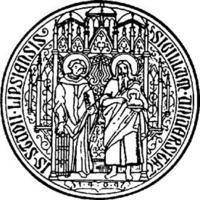
University of Leipzig
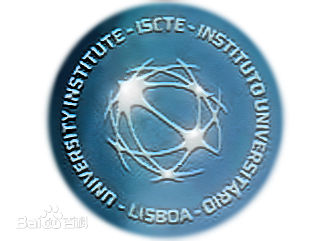
Universidade da Beira Interior
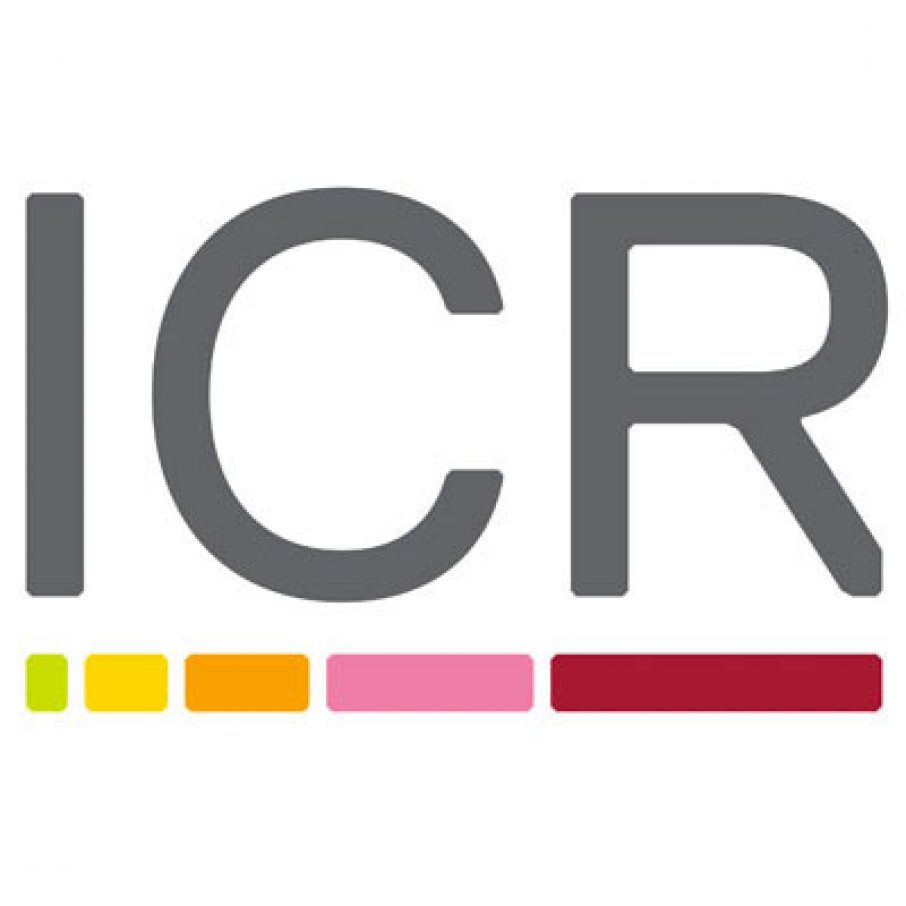
The Institute of Cancer Research
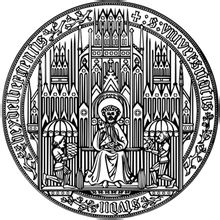
Heidelberg University
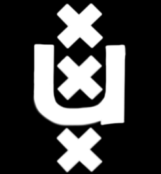
University of Amsterdam
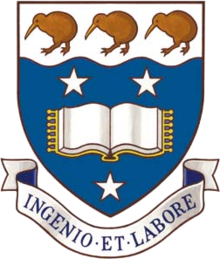
University of Auckland
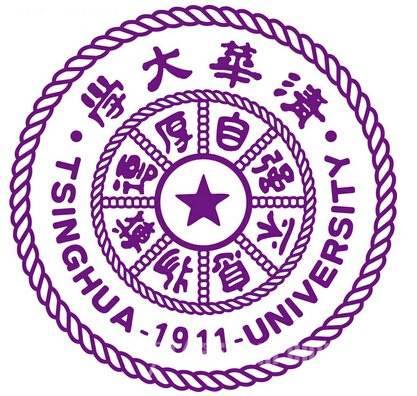
TsingHua University
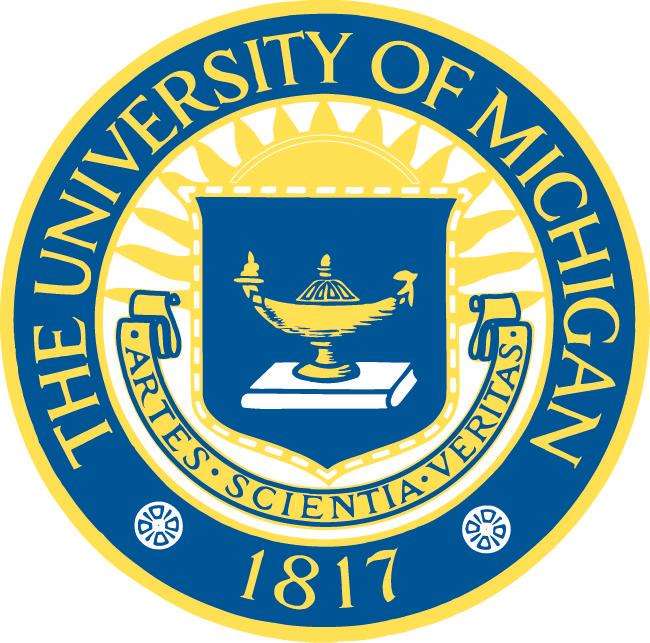
The University of Michigan
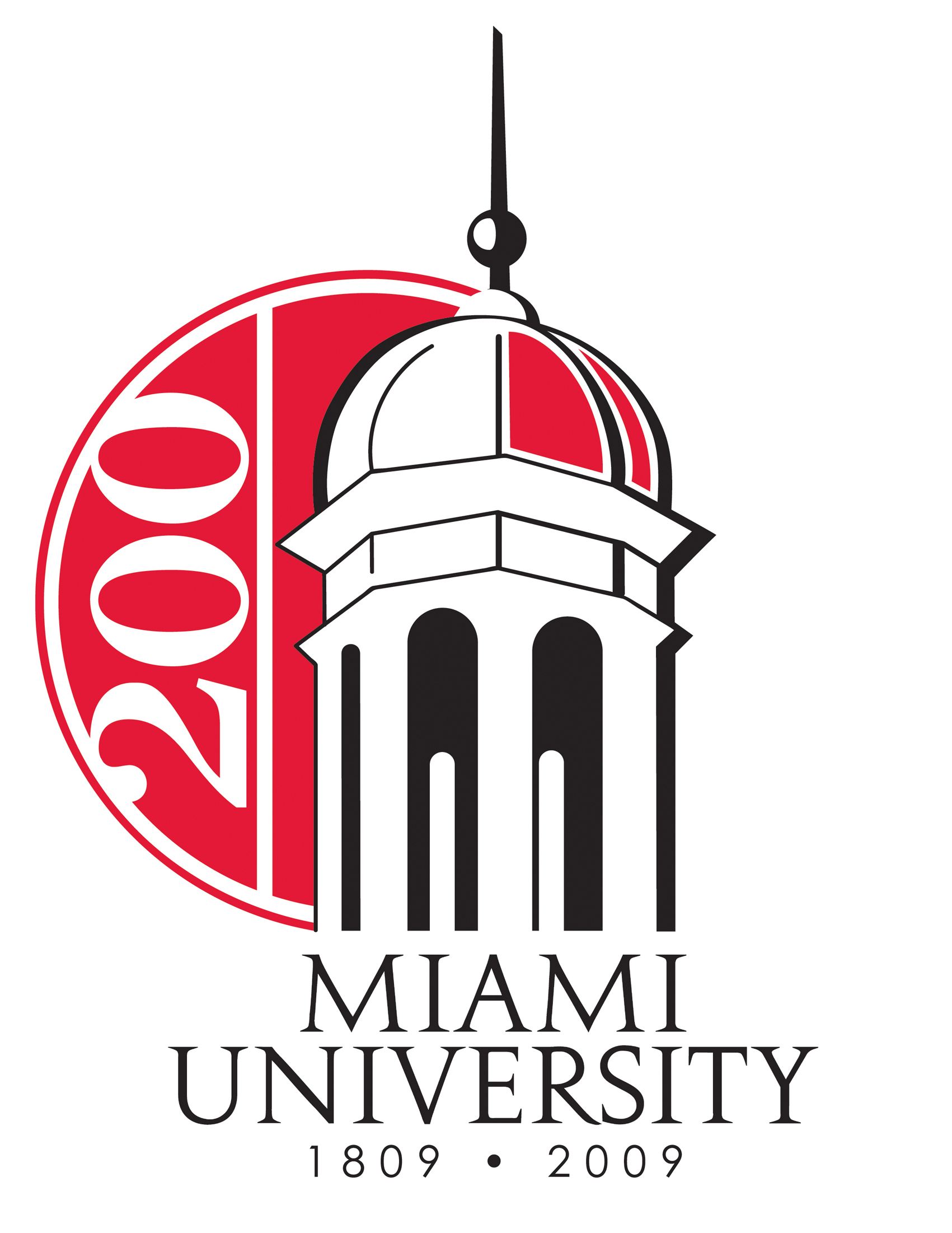
Miami University
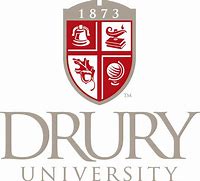
DRURY University
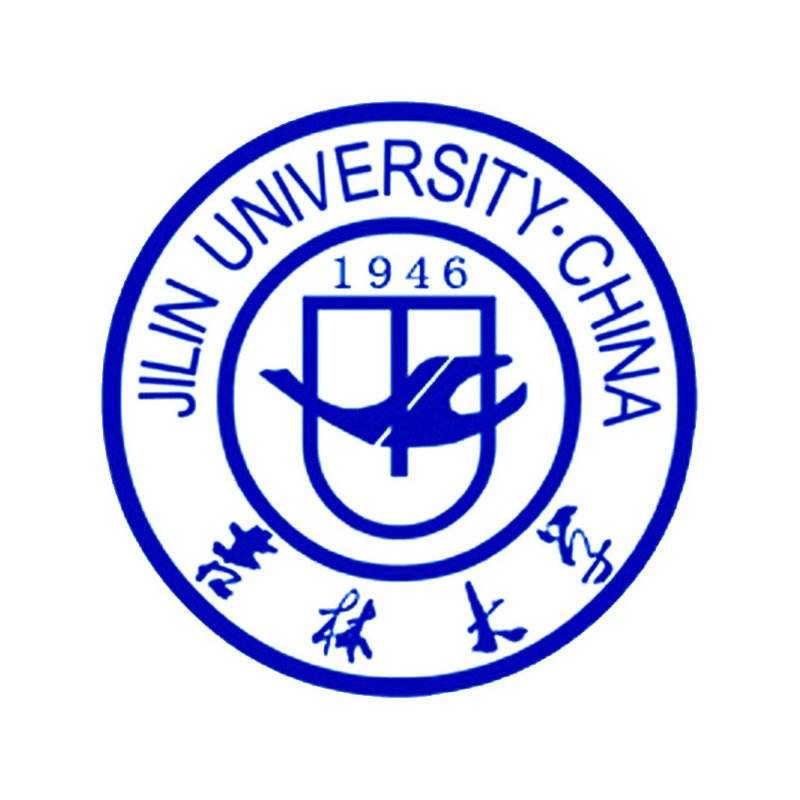
Jilin University
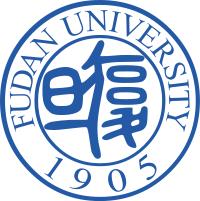
Fudan University
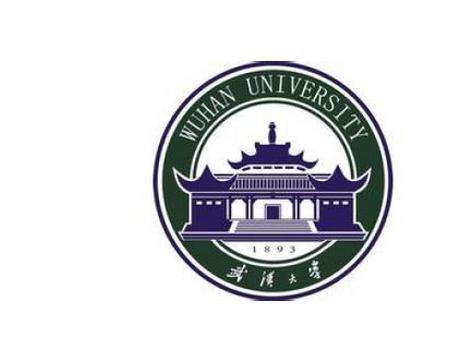
Wuhan University
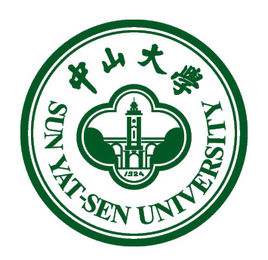
Sun Yat-sen University
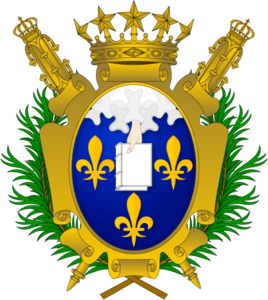
Universite de Paris
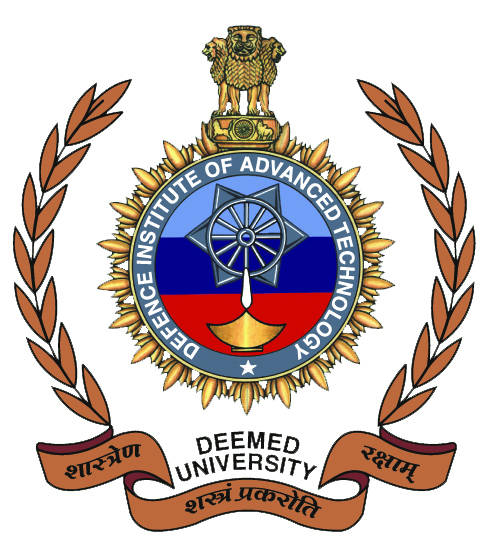
Deemed University
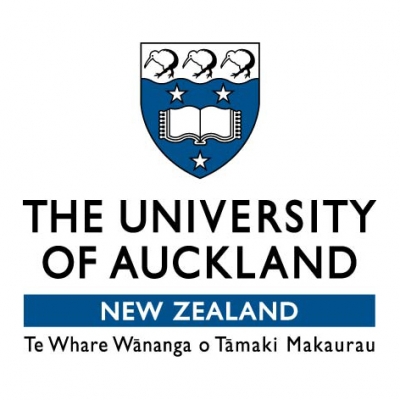
Auckland University
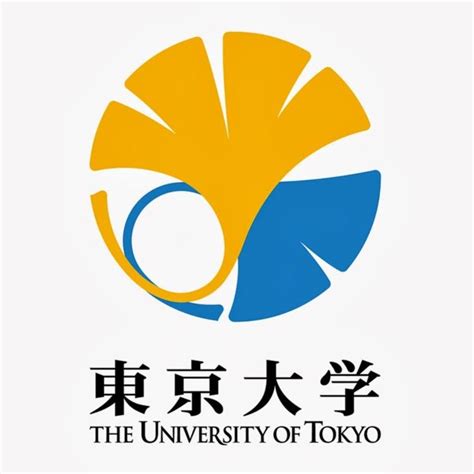
The University of Tokyo
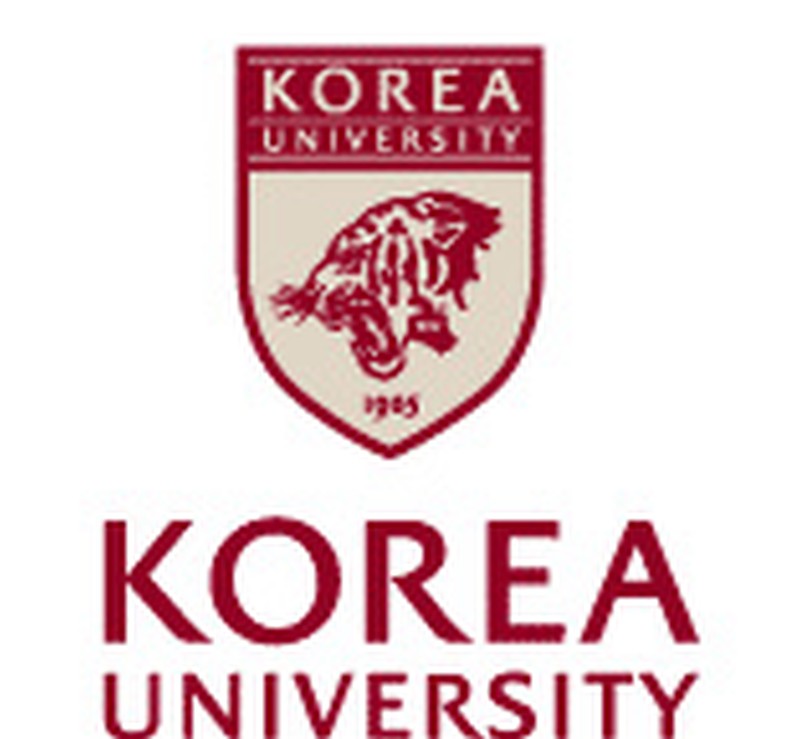
Korea University
- 3-beta-O-(cis-p-Coumaroyl)maslinic acid
Catalog No.:BCN1375
CAS No.:69297-40-1
- 8-O-Acetylharpagide
Catalog No.:BCN4256
CAS No.:6926-14-3
- Harpagide
Catalog No.:BCN4996
CAS No.:6926-08-5
- N-Benzylcinchonidinium chloride
Catalog No.:BCC9094
CAS No.:69257-04-1
- 1,2,3-Tri-O-methyl-7,8-methyleneflavellagic acid
Catalog No.:BCN7205
CAS No.:69251-99-6
- Pinoresinol 4-O-beta-D-glucopyranoside
Catalog No.:BCN1376
CAS No.:69251-96-3
- Osthol hydrate
Catalog No.:BCN1377
CAS No.:69219-24-5
- 2'-Hydroxy-4'-methylacetophenone
Catalog No.:BCN7751
CAS No.:6921-64-8
- (±)-Hexanoylcarnitine chloride
Catalog No.:BCC6680
CAS No.:6920-35-0
- Nesbuvir
Catalog No.:BCC1796
CAS No.:691852-58-1
- (2S,3R,E)-2-Amino-4-heptadecene-1,3-diol
Catalog No.:BCN1765
CAS No.:6918-48-5
- Gomisin N
Catalog No.:BCN2271
CAS No.:69176-52-9
- (R)-baclofen
Catalog No.:BCC4503
CAS No.:69308-37-8
- H-Ile-OtBu.HCl
Catalog No.:BCC2965
CAS No.:69320-89-4
- CYC116
Catalog No.:BCC2181
CAS No.:693228-63-6
- CPPHA
Catalog No.:BCC1501
CAS No.:693288-97-0
- 2-Benzylaminopyridine
Catalog No.:BCC8565
CAS No.:6935-27-9
- Obtucarbamate A
Catalog No.:BCN3936
CAS No.:6935-99-5
- Galanthamine hydrochloride
Catalog No.:BCC8277
CAS No.:5072-47-9
- Boc-D-Thr(Bzl)-OH
Catalog No.:BCC3454
CAS No.:69355-99-3
- Pendulone
Catalog No.:BCN8248
CAS No.:69359-09-7
- Schisanhenol
Catalog No.:BCN2508
CAS No.:69363-14-0
- Sulbactam sodium
Catalog No.:BCC4852
CAS No.:69388-84-7
- Lappaol H
Catalog No.:BCN8415
CAS No.:69394-18-9
Synthesis and pharmaceutical characterization of site specific mycophenolic acid-modified Xenopus glucagon-like peptide-1 analogs.[Pubmed:30108901]
Medchemcomm. 2017 Nov 7;9(1):67-80.
To develop novel long-acting antidiabetic agents, mycophenolic acid (MPA) was used to modify Xenopus glucagon-like peptide-1 analog (GLP-1) (1) at three Lys residues through a gamma-glutamyl linker. Similarly, 6-aminocaproic acid and 12-Aminolauric acid with different lengths of fatty chain were used as MPA derivatives which were then conjugated with 1. By using proper protection and deprotection strategies, the synthetic process was completed directly on the resin to minimize the side reactions, and nine MPA-modified 1 derivatives (2a-2i) were obtained. Compounds 2b and 2c, which showed high GLP-1 receptor activation potencies and glucose lowering activities, were selected for further C-terminal modification to improve their stabilities and bioactivities, giving compounds 3a-3d. The receptor activation potencies and hypoglycemic activities of 3a-3d were comparable to that of liraglutide. Physicochemical and in vitro stability tests revealed that MPA conjugation led to enhanced albumin binding abilities as reflected by the improved stabilities of 3a-3d. In particular, at a dose of 25 nmol kg(-1), the in vivo antidiabetic and insulinotropic activities of 3d were comparable to those of semaglutide. Finally, long-term administration of 3d achieved beneficial effects on glucose tolerance normalization and glycated hemoglobin (HbA1c) lowering, and no hepatotoxicity was observed. In conclusion, this research demonstrated that MPA derivatization was a practical way to develop long-acting antidiabetic peptides.
High yield synthesis of 12-aminolauric acid by "enzymatic transcrystallization" of omega-laurolactam using omega-laurolactam hydrolase from Acidovorax sp. T31.[Pubmed:19420719]
Biosci Biotechnol Biochem. 2009 May;73(5):980-6.
The genes encoding omega-laurolactam hydrolases from Cupriavidus sp. T7, Acidovorax sp. T31, Cupriavidus sp. U124, and Sphingomonas sp. U238 were cloned and sequenced. Nucleotide and amino acid sequence analysis of the four genes indicated that the primary structures of these omega-laurolactam hydrolases are significantly similar to the 6-aminohexanoate-cyclic-dimer hydrolase (EC 3.5.2.12). These genes were expressed in Escherichia coli, and the omega-laurolactam hydrolysing activity of the recombinant enzymes was compared with that of 6-aminohexanoate-cyclic-dimer hydrolase from Arthrobacter sp. KI72. The enzyme from Acidovorax sp. T31 was most successfully expressed in E. coli. Cell-free extract of the recombinant strain was used for the synthesis of 12-Aminolauric acid from omega-laurolactam by "enzymatic transcrystallization," because crystalline omega-laurolactam added into the enzyme solution was converted to crystalline 12-Aminolauric acid (> or =97.3% yield). Under the optimum conditions, 208 g/l of 12-Aminolauric acid was produced in 17 h. The resulting pure product was identical to authentic 12-Aminolauric acid.
The screening, characterization, and use of omega-laurolactam hydrolase: a new enzymatic synthesis of 12-aminolauric acid.[Pubmed:18685217]
Biosci Biotechnol Biochem. 2008 Aug;72(8):2141-50. Epub 2008 Aug 7.
Several omega-laurolactam degrading microorganisms were isolated from soil samples. These strains were capable of growing in a medium containing omega-laurolactam as sole source of carbon and nitrogen. Among them, five strains (T7, T31, U124, U224, and U238) were identified as Cupriavidus sp. T7, Acidovorax sp. T31, Cupriavidus sp. U124, Rhodococcus sp. U224, and Sphingomonas sp. U238, respectively. The omega-laurolactam hydrolyzing enzyme from Rhodococcus sp. U224 was purified to homogeneity, and its enzymatic properties were characterized. The enzyme acts on omega-octalactam and omega-laurolactam, but other lactam compounds, amides and amino acid amides, cannot be substrates. The enzyme gene was cloned, and the deduced amino acid sequence showed high homology with 6-aminohexanoate-cyclic-dimer hydrolase (EC 3.5.2.12) from Arthrobacter sp. KI72 and Pseudomonas sp. NK87. Enzymatic synthesis of 12-Aminolauric acid was performed using partially purified omega-laurolactam hydrolase from Rhodococcus sp. U224.
Molecular interactions alter clay and polymer structure in polymer clay nanocomposites.[Pubmed:18572562]
J Nanosci Nanotechnol. 2008 Apr;8(4):1638-57.
In this work, using photoacoustic Fourier transform infrared spectroscopy (FTIR) we have studied the structural distortion of clay crystal structure in organically modified montmorillonite (OMMT) and polymer clay nanocomposites (PCN). To study the effect of organic modifiers on the distortion of crystal structure of clay, we have synthesized OMMTs and PCNs containing same polymer and clay but with three different organic modifiers (12-Aminolauric acid, n-dodecylamine, and 1,12-diaminododecane), and conducted the FTIR study on these PCNs. Our previous molecular dynamics (MD) study on these PCNs reveals that significant nonbonded interactions (van der Waals, electrostatic interactions) exist between the different constituents (polymer, organic modifier, and clay) of nanocomposites. Previous work based on X-ray diffraction (XRD) and differential scanning calorimetry (DSC) on the same set of PCNs shows that crystallinity of polymer in PCNs have changed significantly in comparison to those in pristine polymer; and, the nonbonded interactions between different constituents of PCN are responsible for the change in crystal structure of polymer in PCN. In this work to evaluate the structural distortion of crystal structure of clay in OMMTs and PCNs, the positions of bands corresponding to different modes of vibration of Si-O bonds are determined from the deconvolution of broad Si-O bands in OMMTs and PCNs obtained from FTIR spectra. Intensity and area under the Si-O bands are indicative of orientation of clay crystal structures in OMMTs and PCNs. Significant changes in the Si-O bands are observed from each vibration mode in OMMTs and PCNs containing three different organic modifiers indicating that organic modifiers influence the structural orientation of silica tetrahedra in OMMTs and PCNs. Deconvolution of Si-O bands in OMMTs indicate a band at approximately 1200 cm(-1) that is orientation-dependent Si-O band. The specific changes in intensity and area under this band for OMMTs with three different organic modifiers further confirm the change in structural orientation of silica tetrahedra of OMMTs by organic modifiers. Thus, from our work it is evident that organic modifiers have significant influence on the structure of polymer and clay in PCNs. It appears that in nanocomposites, in addition to strong interactions at interfaces between constituents, the structure of different phases (clay and polymer) of PCN are also altered, which does not occur in conventional composite materials. Thus, the mechanisms governing composite action in nanocomposites are quite different from that of conventional macro composites.