4-HydroxyquinolineCAS# 611-36-9 |
Quality Control & MSDS
Package In Stock
Number of papers citing our products
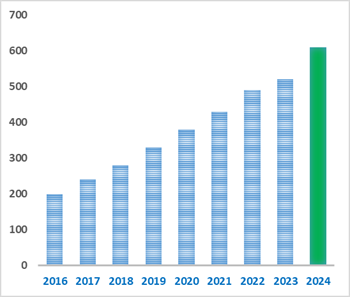
Cas No. | 611-36-9 | SDF | Download SDF |
PubChem ID | N/A | Appearance | Powder |
Formula | C9H7NO | M.Wt | 145.1 |
Type of Compound | Alkaloids | Storage | Desiccate at -20°C |
Solubility | Soluble in Chloroform,Dichloromethane,Ethyl Acetate,DMSO,Acetone,etc. | ||
General tips | For obtaining a higher solubility , please warm the tube at 37 ℃ and shake it in the ultrasonic bath for a while.Stock solution can be stored below -20℃ for several months. We recommend that you prepare and use the solution on the same day. However, if the test schedule requires, the stock solutions can be prepared in advance, and the stock solution must be sealed and stored below -20℃. In general, the stock solution can be kept for several months. Before use, we recommend that you leave the vial at room temperature for at least an hour before opening it. |
||
About Packaging | 1. The packaging of the product may be reversed during transportation, cause the high purity compounds to adhere to the neck or cap of the vial.Take the vail out of its packaging and shake gently until the compounds fall to the bottom of the vial. 2. For liquid products, please centrifuge at 500xg to gather the liquid to the bottom of the vial. 3. Try to avoid loss or contamination during the experiment. |
||
Shipping Condition | Packaging according to customer requirements(5mg, 10mg, 20mg and more). Ship via FedEx, DHL, UPS, EMS or other couriers with RT, or blue ice upon request. |
Description | 4-Hydroxyquinolines exert various antioxidative properties and may thus be used in the development of antioxidant strategies against neurodegenerative diseases associated with oxidative stress. |

4-Hydroxyquinoline Dilution Calculator

4-Hydroxyquinoline Molarity Calculator
1 mg | 5 mg | 10 mg | 20 mg | 25 mg | |
1 mM | 6.8918 mL | 34.459 mL | 68.918 mL | 137.836 mL | 172.295 mL |
5 mM | 1.3784 mL | 6.8918 mL | 13.7836 mL | 27.5672 mL | 34.459 mL |
10 mM | 0.6892 mL | 3.4459 mL | 6.8918 mL | 13.7836 mL | 17.2295 mL |
50 mM | 0.1378 mL | 0.6892 mL | 1.3784 mL | 2.7567 mL | 3.4459 mL |
100 mM | 0.0689 mL | 0.3446 mL | 0.6892 mL | 1.3784 mL | 1.7229 mL |
* Note: If you are in the process of experiment, it's necessary to make the dilution ratios of the samples. The dilution data above is only for reference. Normally, it's can get a better solubility within lower of Concentrations. |
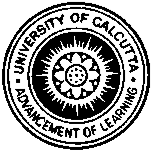
Calcutta University
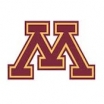
University of Minnesota
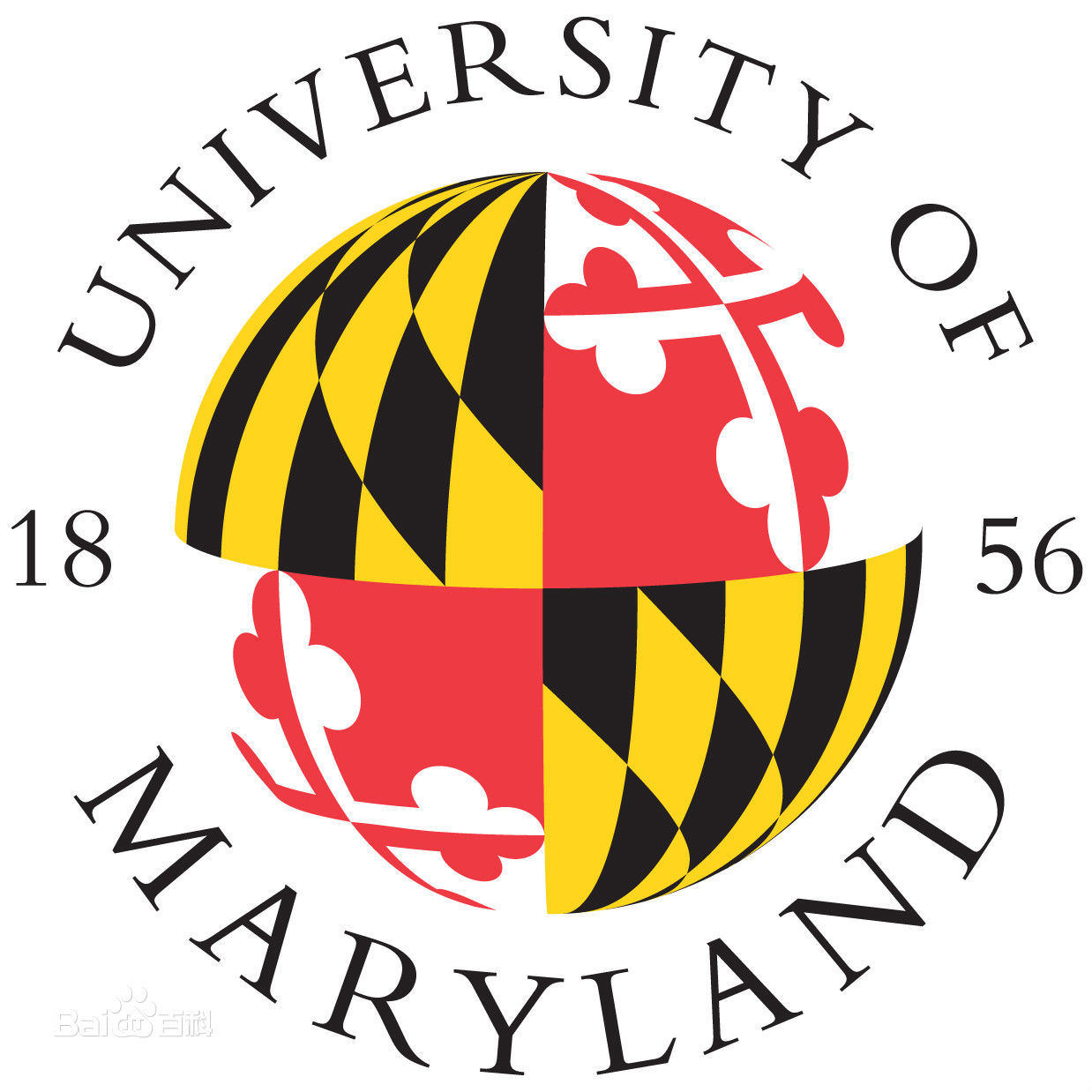
University of Maryland School of Medicine
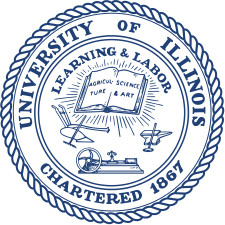
University of Illinois at Chicago
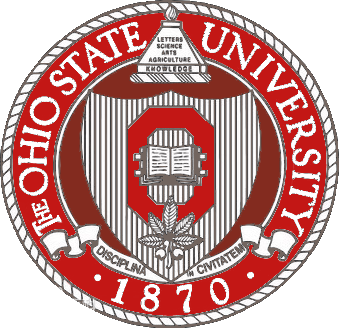
The Ohio State University
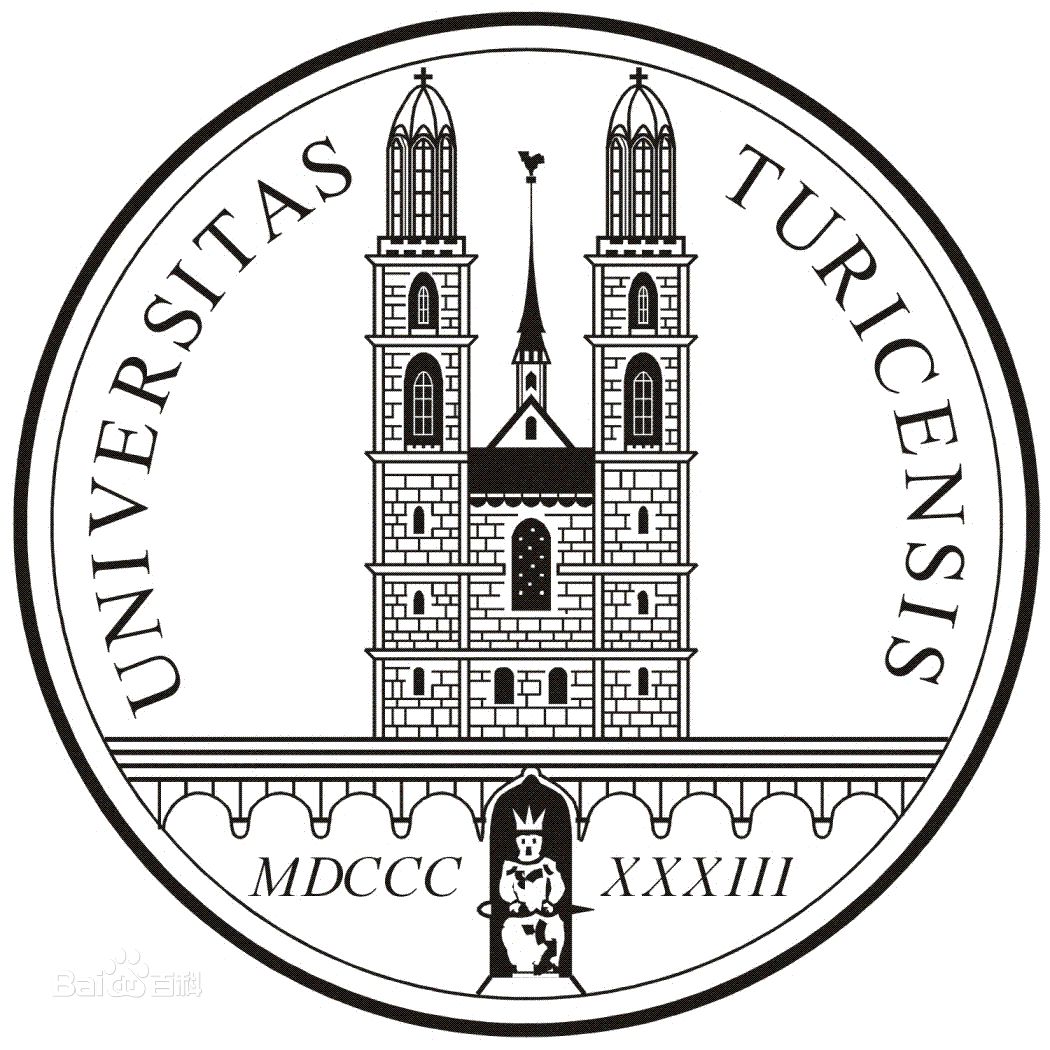
University of Zurich
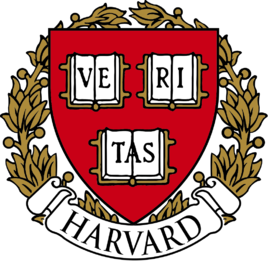
Harvard University
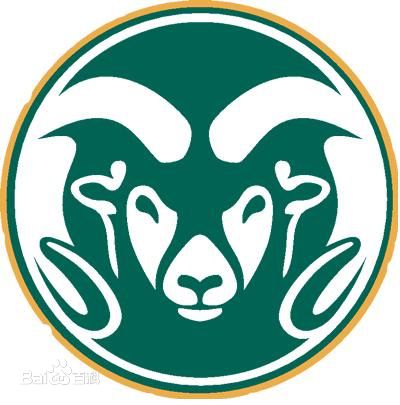
Colorado State University
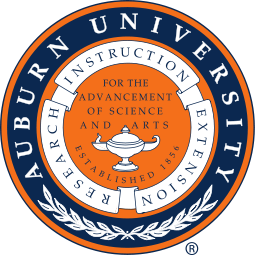
Auburn University
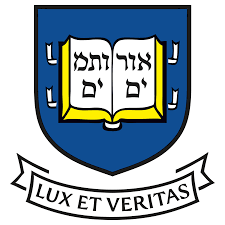
Yale University
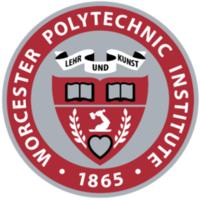
Worcester Polytechnic Institute
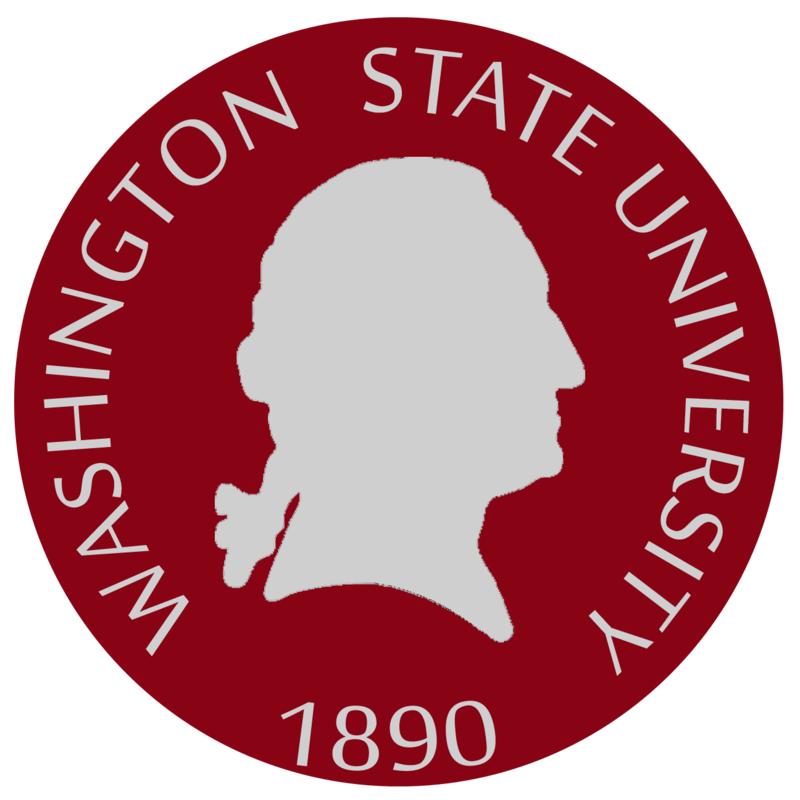
Washington State University
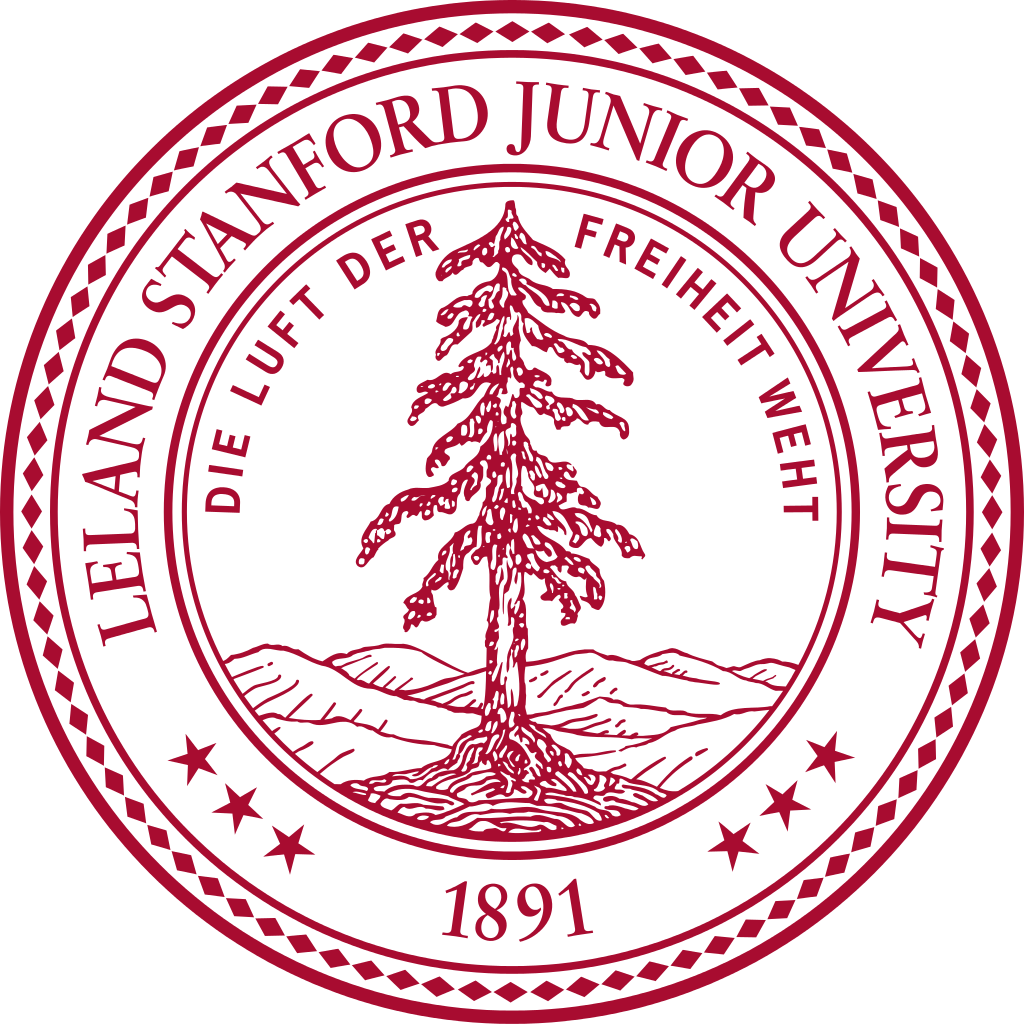
Stanford University
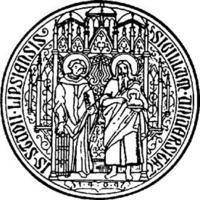
University of Leipzig
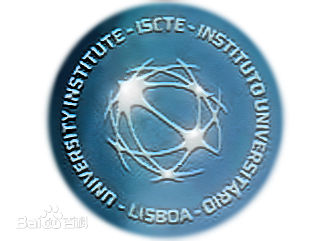
Universidade da Beira Interior
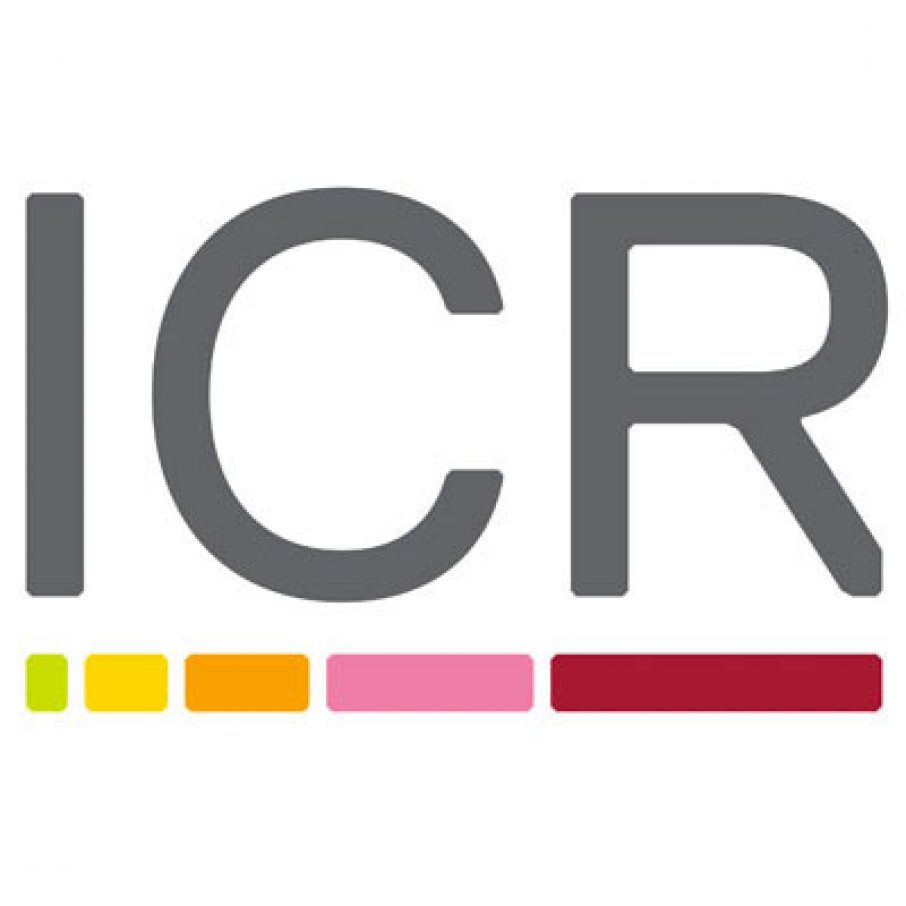
The Institute of Cancer Research
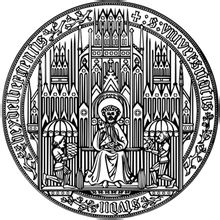
Heidelberg University
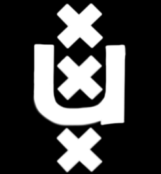
University of Amsterdam
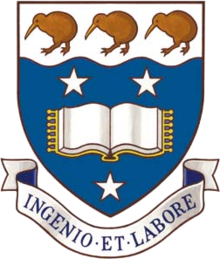
University of Auckland
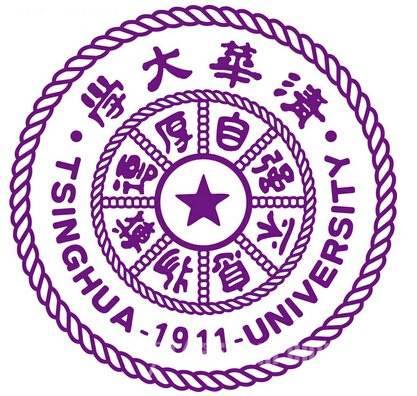
TsingHua University
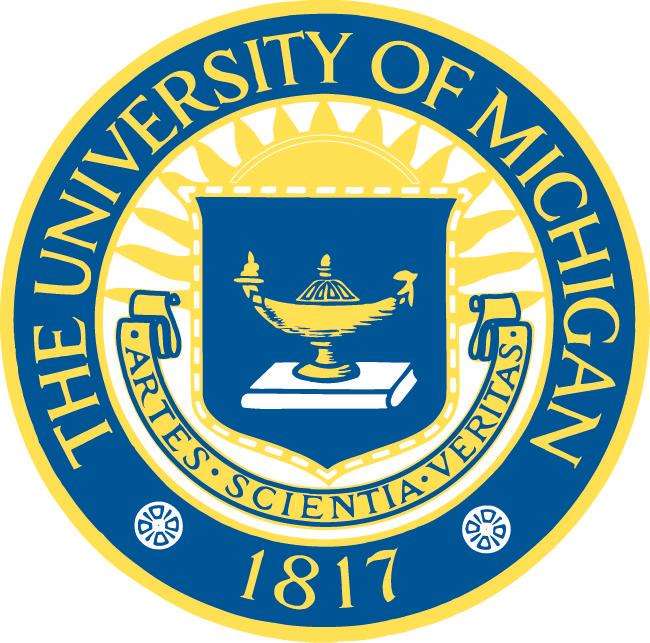
The University of Michigan
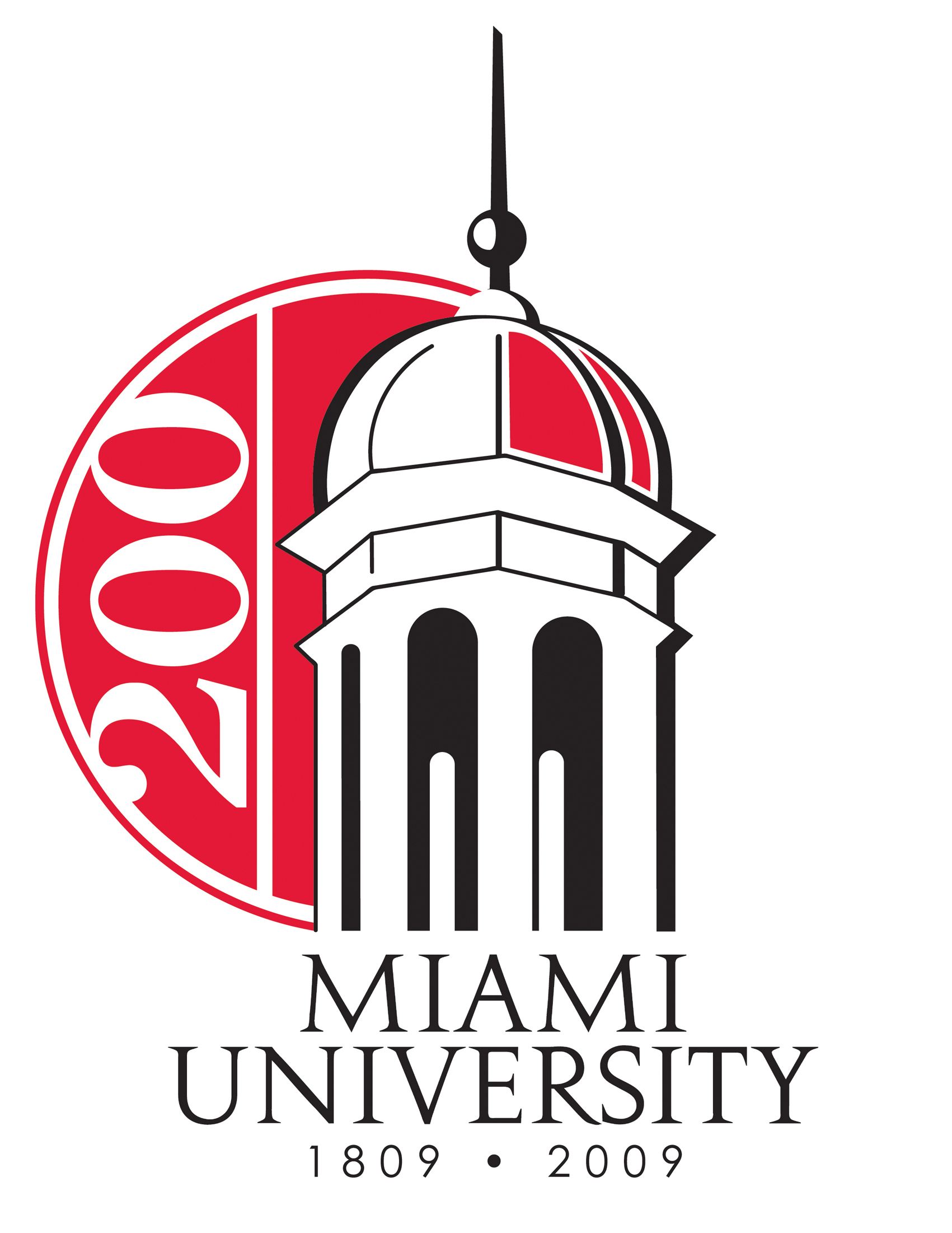
Miami University
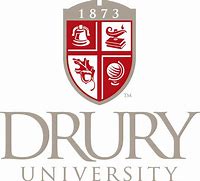
DRURY University
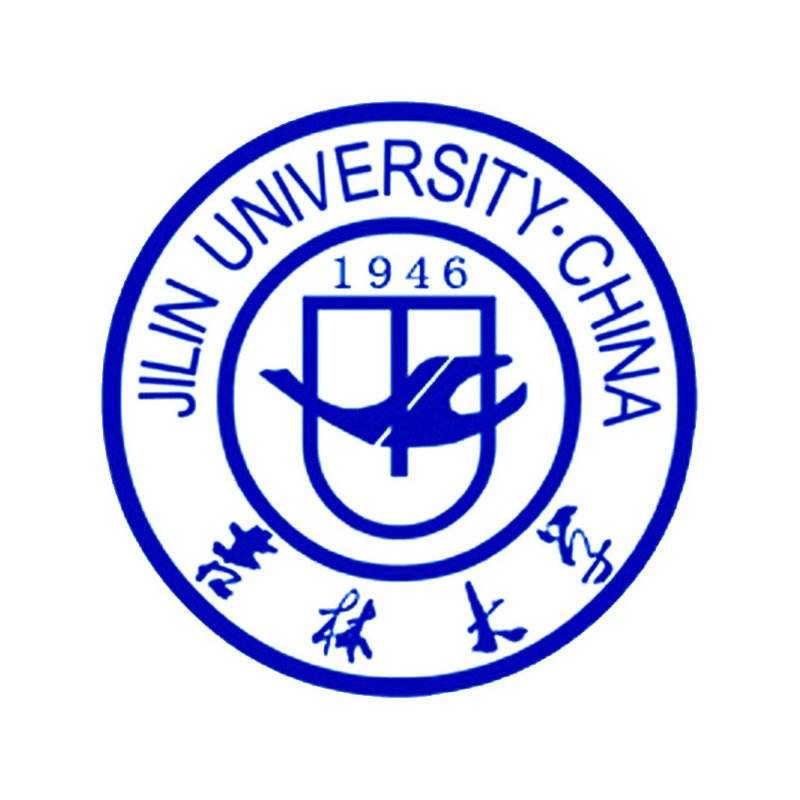
Jilin University
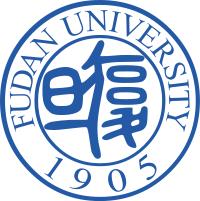
Fudan University
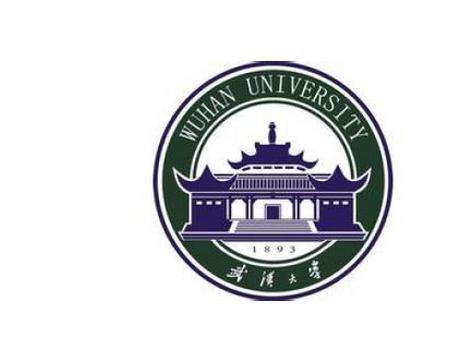
Wuhan University
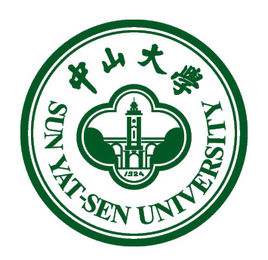
Sun Yat-sen University
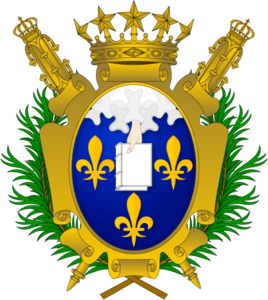
Universite de Paris
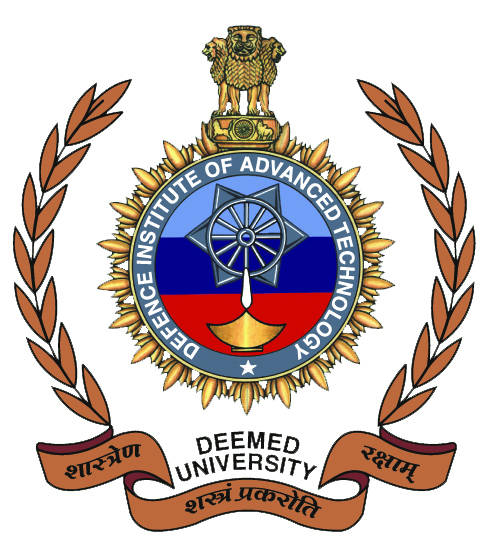
Deemed University
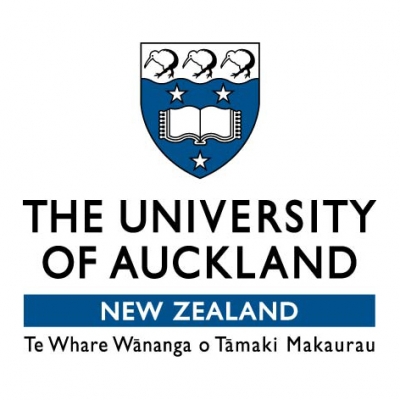
Auckland University
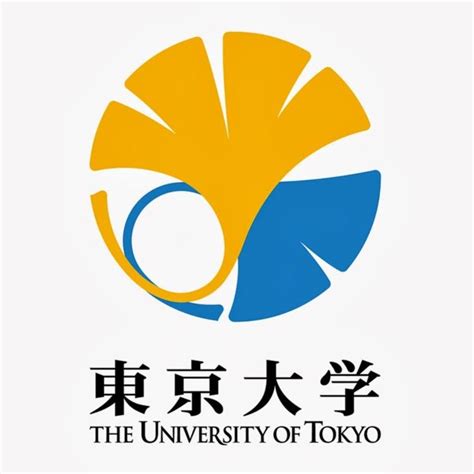
The University of Tokyo
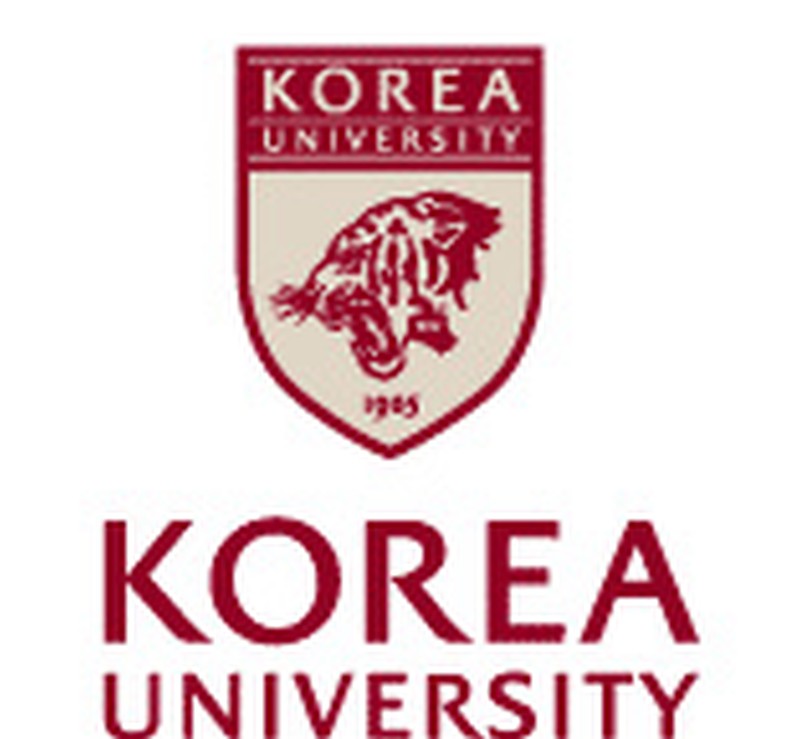
Korea University
- Cytochalasin C
Catalog No.:BCN9993
CAS No.:22144-76-9
- Geranylacetate
Catalog No.:BCN9992
CAS No.:105-87-3
- Daclatasvir
Catalog No.:BCN9991
CAS No.:1009119-64-5
- Phloroglucinol aldehyde triethylether
Catalog No.:BCN9990
CAS No.:59652-88-9
- 3-Octyl alcohol
Catalog No.:BCN9989
CAS No.:589-98-0
- trans-Aconitic acid
Catalog No.:BCN9988
CAS No.:4023-65-8
- Quercetin-3'-glucoside
Catalog No.:BCN9987
CAS No.:19254-30-9
- 1,3-Diphenyl-2-propen-1-one
Catalog No.:BCN9986
CAS No.:94-41-7
- 4'-Methoxyflavanone
Catalog No.:BCN9985
CAS No.:97005-76-0
- Farnesol
Catalog No.:BCN9984
CAS No.:4602-84-0
- (R,S)-Equol
Catalog No.:BCN9983
CAS No.:66036-38-2
- 4',6,7-Trimethoxyisoflavone
Catalog No.:BCN9982
CAS No.:798-61-8
- 2',3,5,7-Tetrahydroxyflavone
Catalog No.:BCN9995
CAS No.:480-15-9
- 3,4-Dimethoxychalcone
Catalog No.:BCN9996
CAS No.:5416-71-7
- Oxyacanthine hydrochloride
Catalog No.:BCN9997
CAS No.:15352-74-6
- 7-Methoxyflavone
Catalog No.:BCN9998
CAS No.:22395-22-8
- trans-5-Hydroxyferulic acid
Catalog No.:BCN9999
CAS No.:110642-42-7
- 8-p-Coumaroylharpagide
Catalog No.:BCN0152
CAS No.:87686-74-6
- 2-(4-Methoxybenzal)acetophenone
Catalog No.:BCN0001
CAS No.:959-33-1
- N-Methylcolchicine
Catalog No.:BCN0002
CAS No.:7336-40-5
- Hydrocotarnine hydrobromide
Catalog No.:BCN0003
CAS No.:5985-00-2
- 8-Acetyl-7-methoxycoumarin
Catalog No.:BCN0004
CAS No.:89019-07-8
- Shatavarin IV
Catalog No.:BCN0005
CAS No.:84633-34-1
- N-trans-p-Coumaroyltyrosine
Catalog No.:BCN0006
CAS No.:77201-66-2
Modification of the Pseudomonas aeruginosa toxin 2-heptyl-1-hydroxyquinolin-4(1H)-one and other secondary metabolites by methyltransferases from mycobacteria.[Pubmed:33064871]
FEBS J. 2020 Oct 16.
The opportunistic pathogen Pseudomonas aeruginosa, one of the most prevalent species in infections of the cystic fibrosis lung, produces a range of secondary metabolites, among them the respiratory toxin 2-heptyl-1-hydroxyquinolin-4(1H)-one (2-heptyl-4-Hydroxyquinoline N-oxide, HQNO). Cultures of the emerging cystic fibrosis pathogen Mycobacteroides abscessus detoxify HQNO by methylating the N-hydroxy moiety. In this study, the class I methyltransferase MAB_2834c and its orthologue from Mycobacterium tuberculosis, Rv0560c, were identified as HQNO O-methyltransferases. The P. aeruginosa exoproducts 4-hydroxyquinolin-2(1H)-one (DHQ), 2-heptylquinolin-4(1H)-one (HHQ), and 2-heptyl-3-hydroxyquinolin-4(1H)-one (the 'Pseudomonas quinolone signal', PQS), some structurally related (iso)quinolones, and the flavonol quercetin were also methylated; however, HQNO was by far the preferred substrate. Both enzymes converted a benzimidazole[1,2-a]pyridine-4-carbonitrile-based compound, representing the scaffold of antimycobacterial substances, to an N-methylated derivative. We suggest that these promiscuous methyltransferases, newly termed as heterocyclic toxin methyltransferases (Htm), are involved in cellular response to chemical stress and possibly contribute to resistance of mycobacteria toward antimicrobial natural compounds as well as drugs. Thus, synthetic antimycobacterial agents may be designed to be unamenable to methyl transfer. ENZYMES: S-adenosyl-l-methionine:2-heptyl-1-hydroxyquinolin-4(1H)-one O-methyl-transferase, EC 2.1.1.
Spatiotemporal Distribution of Pseudomonas aeruginosa Alkyl Quinolones under Metabolic and Competitive Stress.[Pubmed:32699119]
mSphere. 2020 Jul 22;5(4). pii: 5/4/e00426-20.
Pseudomonas aeruginosa is an opportunistic human pathogen important to diseases such as cystic fibrosis. P. aeruginosa has multiple quorum-sensing (QS) systems, one of which utilizes the signaling molecule 2-heptyl-3-hydroxy-4-quinolone (Pseudomonas quinolone signal [PQS]). Here, we use hyperspectral Raman imaging to elucidate the spatiotemporal PQS distributions that determine how P. aeruginosa regulates surface colonization and its response to both metabolic stress and competition from other bacterial strains. These chemical imaging experiments illustrate the strong link between environmental challenges, such as metabolic stress caused by nutritional limitations or the presence of another bacterial species, and PQS signaling. Metabolic stress elicits a complex response in which limited nutrients induce the bacteria to produce PQS earlier, but the bacteria may also pause PQS production entirely if the nutrient concentration is too low. Separately, coculturing P. aeruginosa in the proximity of another bacterial species, or its culture supernatant, results in earlier production of PQS. However, these differences in PQS appearance are not observed for all alkyl quinolones (AQs) measured; the spatiotemporal response of 2-heptyl-4-Hydroxyquinoline N-oxide (HQNO) is highly uniform for most conditions. These insights on the spatiotemporal distributions of quinolones provide additional perspective on the behavior of P. aeruginosa in response to different environmental cues.IMPORTANCE Alkyl quinolones (AQs), including Pseudomonas quinolone signal (PQS), made by the opportunistic pathogen Pseudomonas aeruginosa have been associated with both population density and stress. The regulation of AQ production is known to be complex, and the stimuli that modulate AQ responses are not fully clear. Here, we have used hyperspectral Raman chemical imaging to examine the temporal and spatial profiles of AQs exhibited by P. aeruginosa under several potentially stressful conditions. We found that metabolic stress, effected by carbon limitation, or competition stress, effected by proximity to other species, resulted in accelerated PQS production. This competition effect did not require cell-to-cell interaction, as evidenced by the fact that the addition of supernatants from either Escherichia coli or Staphylococcus aureus led to early appearance of PQS. Lastly, the fact that these modulations were observed for PQS but not for all AQs suggests a high level of complexity in AQ regulation that remains to be discerned.
Pseudomonas aeruginosa PA14 Enhances the Efficacy of Norfloxacin against Staphylococcus aureus Newman Biofilms.[Pubmed:32661077]
J Bacteriol. 2020 Aug 25;202(18). pii: JB.00159-20.
The thick mucus within the airways of individuals with cystic fibrosis (CF) promotes frequent respiratory infections that are often polymicrobial. Pseudomonas aeruginosa and Staphylococcus aureus are two of the most prevalent pathogens that cause CF pulmonary infections, and both are among the most common etiologic agents of chronic wound infections. Furthermore, the ability of P. aeruginosa and S. aureus to form biofilms promotes the establishment of chronic infections that are often difficult to eradicate using antimicrobial agents. In this study, we found that multiple LasR-regulated exoproducts of P. aeruginosa, including 2-heptyl-4-Hydroxyquinoline N-oxide (HQNO), siderophores, phenazines, and rhamnolipids, likely contribute to the ability of P. aeruginosa PA14 to shift S. aureus Newman norfloxacin susceptibility profiles. Here, we observe that exposure to P. aeruginosa exoproducts leads to an increase in intracellular norfloxacin accumulation by S. aureus We previously showed that P. aeruginosa supernatant dissipates the S. aureus membrane potential, and furthermore, depletion of the S. aureus proton motive force recapitulates the effect of the P. aeruginosa PA14 supernatant on shifting norfloxacin sensitivity profiles of biofilm-grown S. aureus Newman. From these results, we hypothesize that exposure to P. aeruginosa PA14 exoproducts leads to increased uptake of the drug and/or an impaired ability of S. aureus Newman to efflux norfloxacin. Surprisingly, the effect observed here of P. aeruginosa PA14 exoproducts on S. aureus Newman susceptibility to norfloxacin seemed to be specific to these strains and this antibiotic. Our results illustrate that microbially derived products can alter the ability of antimicrobial agents to kill bacterial biofilms.IMPORTANCE Pseudomonas aeruginosa and Staphylococcus aureus are frequently coisolated from multiple infection sites, including the lungs of individuals with cystic fibrosis (CF) and nonhealing diabetic foot ulcers. Coinfection with P. aeruginosa and S. aureus has been shown to produce worse outcomes compared to infection with either organism alone. Furthermore, the ability of these pathogens to form biofilms enables them to cause persistent infection and withstand antimicrobial therapy. In this study, we found that P. aeruginosa-secreted products dramatically increase the ability of the antibiotic norfloxacin to kill S. aureus biofilms. Understanding how interspecies interactions alter the antibiotic susceptibility of bacterial biofilms may inform treatment decisions and inspire the development of new therapeutic strategies.
Pseudomonas Quinolone Signal molecule PQS behaves like a B Class inhibitor at the IQ site of mitochondrial complex I.[Pubmed:32161908]
FASEB Bioadv. 2020 Feb 19;2(3):188-202.
Pseudomonas aeruginosa is a Gram-negative bacterium of the proteobacteria class, and one of the most common causes of nosocomial infections. For example, it causes chronic pneumonia in cystic fibrosis patients. Patient sputum contains 2-heptyl-4-Hydroxyquinoline N-oxide [HQNO] and Pseudomonas quorum sensing molecules such as the Pseudomonas quinolone signal [PQS]. It is known that HQNO inhibits the enzyme activity of mitochondrial and bacterial complex III at the Qi (quinone reduction) site, but the target of PQS is not known. In this work we have shown that PQS has a negative effect on mitochondrial respiration in HeLa and A549 cells. It specifically inhibits the complex I of the respiratory chain. In vitro analyses showed a partially competitive inhibition with respect to ubiquinone at the IQ site. In competing studies with Rotenone, PQS suppressed the ROS-promoting effect of Rotenone, which is typical for a B-type inhibitor. Prolonged incubation with PQS also had an effect on the activity of complex III.
Structure of the cytochrome aa 3 -600 heme-copper menaquinol oxidase bound to inhibitor HQNO shows TM0 is part of the quinol binding site.[Pubmed:31888984]
Proc Natl Acad Sci U S A. 2020 Jan 14;117(2):872-876.
Virtually all proton-pumping terminal respiratory oxygen reductases are members of the heme-copper oxidoreductase superfamily. Most of these enzymes use reduced cytochrome c as a source of electrons, but a group of enzymes have evolved to directly oxidize membrane-bound quinols, usually menaquinol or ubiquinol. All of the quinol oxidases have an additional transmembrane helix (TM0) in subunit I that is not present in the related cytochrome c oxidases. The current work reports the 3.6-A-resolution X-ray structure of the cytochrome aa 3 -600 menaquinol oxidase from Bacillus subtilis containing 1 equivalent of menaquinone. The structure shows that TM0 forms part of a cleft to accommodate the menaquinol-7 substrate. Crystals which have been soaked with the quinol-analog inhibitor HQNO (N-oxo-2-heptyl-4-Hydroxyquinoline) or 3-iodo-HQNO reveal a single binding site where the inhibitor forms hydrogen bonds to amino acid residues shown previously by spectroscopic methods to interact with the semiquinone state of menaquinone, a catalytic intermediate.
Unprecedented Properties of Phenothiazines Unraveled by a NDH-2 Bioelectrochemical Assay Platform.[Pubmed:31880924]
J Am Chem Soc. 2020 Jan 22;142(3):1311-1320.
Type II NADH:quinone oxidoreductase (NDH-2) plays a crucial role in the respiratory chains of many organisms. Its absence in mammalian cells makes NDH-2 an attractive new target for developing antimicrobials and antiprotozoal agents. We established a novel bioelectrochemical platform to characterize the catalytic behavior of NDH-2 from Caldalkalibacillus thermarum and Listeria monocytogenes strain EGD-e while bound to native-like lipid membranes. Catalysis of both NADH oxidation and lipophilic quinone reduction by membrane-bound NDH-2 followed the Michaelis-Menten model; however, the maximum turnover was only achieved when a high concentration of quinone (>3 mM) was present in the membrane, suggesting that quinone availability regulates NADH-coupled respiration activity. The quinone analogue 2-heptyl-4-Hydroxyquinoline-N-oxide inhibited C. thermarum NDH-2 activity, and its potency is higher in a membrane environment compared to assays performed with water-soluble quinone analogues, demonstrating the importance of testing compounds under physiologically relevant conditions. Furthermore, when phenothiazines, one of the most commonly identified NDH-2 inhibitors, were tested, they did not inhibit membrane-bound NDH-2. Instead, our assay platform unexpectedly suggests a novel mode of phenothiazine action where chlorpromazine, a promising antitubercular agent and key medicine used to treat psychotic disorders, is able to disrupt pH gradients across bacterial membranes.
Copper-Catalyzed Chemoselective Cyclization Reaction of 2-Isocyanoacetophenone: Synthesis of 4-Hydroxyquinoline Compounds.[Pubmed:31825222]
J Org Chem. 2020 Jan 17;85(2):1279-1284.
A copper-catalyzed intramolecular cyclization reaction of 2-isocyanoacetophenone derivatives to afford 4-Hydroxyquinolines chemoselectively is described. The transformation proceeds through enol tautomerism and a subsequent C-C bond formation. Compared to previous methods, this study provides a new protocol for the construction of 4-Hydroxyquinoline compounds from functionalized isocyanides under mild conditions.
Exogenous Alginate Protects Staphylococcus aureus from Killing by Pseudomonas aeruginosa.[Pubmed:31792010]
J Bacteriol. 2020 Mar 26;202(8). pii: JB.00559-19.
Cystic fibrosis (CF) patients chronically infected with both Pseudomonas aeruginosa and Staphylococcus aureus have worse health outcomes than patients who are monoinfected with either P. aeruginosa or S. aureus We showed previously that mucoid strains of P. aeruginosa can coexist with S. aureus in vitro due to the transcriptional downregulation of several toxic exoproducts typically produced by P. aeruginosa, including siderophores, rhamnolipids, and HQNO (2-heptyl-4-Hydroxyquinoline N-oxide). Here, we demonstrate that exogenous alginate protects S. aureus from P. aeruginosa in both planktonic and biofilm coculture models under a variety of nutritional conditions. S. aureus protection in the presence of exogenous alginate is due to the transcriptional downregulation of pvdA, a gene required for the production of the iron-scavenging siderophore pyoverdine as well as the downregulation of the PQS (Pseudomonas quinolone signal) (2-heptyl-3,4-dihydroxyquinoline) quorum sensing system. The impact of exogenous alginate is independent of endogenous alginate production. We further demonstrate that coculture of mucoid P. aeruginosa with nonmucoid P. aeruginosa strains can mitigate the killing of S. aureus by the nonmucoid strain of P. aeruginosa, indicating that the mechanism that we describe here may function in vivo in the context of mixed infections. Finally, we investigated a panel of mucoid clinical isolates that retain the ability to kill S. aureus at late time points and show that each strain has a unique expression profile, indicating that mucoid isolates can overcome the S. aureus-protective effects of mucoidy in a strain-specific manner.IMPORTANCE CF patients are chronically infected by polymicrobial communities. The two dominant bacterial pathogens that infect the lungs of CF patients are P. aeruginosa and S. aureus, with approximately 30% of patients coinfected by both species. Such coinfected individuals have worse outcomes than monoinfected patients, and both species persist within the same physical space. A variety of host and environmental factors have been demonstrated to promote P. aeruginosa-S. aureus coexistence, despite evidence that P. aeruginosa kills S. aureus when these organisms are cocultured in vitro Thus, a better understanding of P. aeruginosa-S. aureus interactions, particularly mechanisms by which these microorganisms are able to coexist in proximal physical space, will lead to better-informed treatments for chronic polymicrobial infections.
Pseudomonas aeruginosa Increases the Sensitivity of Biofilm-Grown Staphylococcus aureus to Membrane-Targeting Antiseptics and Antibiotics.[Pubmed:31363032]
mBio. 2019 Jul 30;10(4). pii: mBio.01501-19.
Pseudomonas aeruginosa and Staphylococcus aureus often cause chronic, recalcitrant infections in large part due to their ability to form biofilms. The biofilm mode of growth enables these organisms to withstand antibacterial insults that would effectively eliminate their planktonic counterparts. We found that P. aeruginosa supernatant increased the sensitivity of S. aureus biofilms to multiple antimicrobial compounds, including fluoroquinolones and membrane-targeting antibacterial agents, including the antiseptic chloroxylenol. Treatment of S. aureus with the antiseptic chloroxylenol alone did not decrease biofilm cell viability; however, the combination of chloroxylenol and P. aeruginosa supernatant led to a 4-log reduction in S. aureus biofilm viability compared to exposure to chloroxylenol alone. We found that the P. aeruginosa-produced small molecule 2-n-heptyl-4-Hydroxyquinoline N-oxide (HQNO) is responsible for the observed heightened sensitivity of S. aureus to chloroxylenol. Similarly, HQNO increased the susceptibility of S. aureus biofilms to other compounds, including both traditional and nontraditional antibiotics, which permeabilize bacterial membranes. Genetic and phenotypic studies support a model whereby HQNO causes an increase in S. aureus membrane fluidity, thereby improving the efficacy of membrane-targeting antiseptics and antibiotics. Importantly, our data show that P. aeruginosa exoproducts can enhance the ability of various antimicrobial agents to kill biofilm populations of S. aureus that are typically difficult to eradicate. Finally, our discovery that altering membrane fluidity shifts antimicrobial sensitivity profiles of bacterial biofilms may guide new approaches to target persistent infections, such as those commonly found in respiratory tract infections and in chronic wounds.IMPORTANCE The thick mucus in the airways of cystic fibrosis (CF) patients predisposes them to frequent, polymicrobial respiratory infections. Pseudomonas aeruginosa and Staphylococcus aureus are frequently coisolated from the airways of individuals with CF, as well as from diabetic foot ulcers and other wounds. Both organisms form biofilms, which are notoriously difficult to eradicate and promote chronic infection. In this study, we have shown that P. aeruginosa-secreted factors can increase the efficacy of compounds that alone have little or no bactericidal activity against S. aureus biofilms. In particular, we discovered that P. aeruginosa exoproducts can potentiate the antistaphylococcal activity of phenol-based antiseptics and other membrane-active drugs. Our findings illustrate that polymicrobial interactions can dramatically increase antibacterial efficacy in vitro and suggest that altering membrane physiology promotes the ability of certain drugs to kill bacterial biofilms-knowledge that may provide a path for the discovery of new biofilm-targeting antimicrobial strategies.
Tautomeric polymorphism of the neuroactive inhibitor kynurenic acid.[Pubmed:31166934]
Acta Crystallogr C Struct Chem. 2019 Jun 1;75(Pt 6):793-805.
Kynurenic acid (KYN; systematic name: 4-Hydroxyquinoline-2-carboxylic acid, C10H7NO3) displays a therapeutic effect in the treatment of some neurological diseases and is used as a broad-spectrum neuroprotective agent. However, it is understudied with respect to its solid-state chemistry and only one crystal form (alpha-KYN.H2O) has been reported up to now. Therefore, an attempt to synthesize alternative solid-state forms of KYN was undertaken and six new species were obtained: five solvates and one salt. One of them is a new polymorph, beta-KYN.H2O, of the already known KYN monohydrate. All crystal species were further studied by single-crystal and powder X-ray diffraction, thermal and spectroscopic methods. In addition to the above methods, differential scanning calorimetry (DSC), in-situ variable-temperature powder X-ray diffraction and Raman microscopy were applied to characterize the phase behaviour of the new forms. All the compounds display a zwitterionic form of KYN and two different enol-keto tautomers are observed depending on the crystallization solvent used.
2-Alkyl-4-hydroxyquinolines from a Marine-Derived Streptomyces sp. Inhibit Hyphal Growth Induction in Candida albicans.[Pubmed:30813382]
Mar Drugs. 2019 Feb 22;17(2). pii: md17020133.
Four 2-alkyl-4-Hydroxyquinoline derivatives (1(-)4) were isolated from a semisolid rice culture of the marine-derived actinomycete Streptomyces sp. MBTG13. The structures of these compounds were elucidated by a combination of spectroscopic methods, and their data were in good agreement with previous reports. Compounds 1 and 2 exhibited weak to moderate antibacterial activity against pathogenic bacteria. Unexpectedly, we found that compound 1 acted as a potent inhibitor of hyphal growth induction in the dimorphic fungus Candida albicans, with an IC50 value of 11.4 mug/mL. Growth experiments showed that this compound did not inhibit yeast cell growth, but inhibited hyphal growth induction. Semi-quantitative reverse transcription (RT)-PCR analysis of hyphal-inducing signaling pathway components indicated that compound 1 inhibited the expression of mRNAs related to the cAMP-Efg1 pathway. The expression of HWP1 and ALS3 mRNAs (hypha-specific genes positively regulated by Efg1, an important regulator of cell wall dynamics) was significantly inhibited by the addition of compound 1. These results indicate that compound 1 acts on the Efg1-mediated cAMP pathway and regulates hyphal growth in Candida albicans.
Selectivity of Dietary Phenolics for Inhibition of Human Monoamine Oxidases A and B.[Pubmed:30809547]
Biomed Res Int. 2019 Jan 23;2019:8361858.
Monoamine oxidases (MAOs) regulate local levels of neurotransmitters such as dopamine, norepinephrine, and serotonin and thus have been targeted by drugs for the treatment of certain CNS disorders. However, recent studies have shown that these enzymes are upregulated with age in nervous and cardiac tissues and may be involved in degeneration of these tissues, since their metabolic mechanism releases hydrogen peroxide leading to oxidative stress. Thus, targeting these enzymes may be a potential anti-aging strategy. The purpose of this study was to compare the MAO inhibition and selectivity of selected dietary phenolic compounds, using a previously validated assay that would avoid interference from the compounds. Kynuramine metabolism by human recombinant MAO-A and MAO-B leads to formation of 4-Hydroxyquinoline, with Vmax values of 10.2+/-0.2 and 7.35+/-0.69 nmol/mg/min, respectively, and Km values of 23.1+/-0.8 muM and 18.0+/-2.3 muM, respectively. For oral dosing and interactions with the gastrointestinal tract, curcumin, guaiacol, isoeugenol, pterostilbene, resveratrol, and zingerone were tested at their highest expected luminal concentrations from an oral dose. Each of these significantly inhibited both enzymes except for zingerone, which only inhibited MAO-A. The IC50 values were determined, and selectivity indices (MAO-A/MAO-B IC50 ratios) were calculated. Resveratrol and isoeugenol were selective for MAO-A, with IC50 values of 0.313+/-0.008 and 3.72+/-0.20 muM and selectivity indices of 50.5 and 27.4, respectively. Pterostilbene was selective for MAO-B, with IC50 of 0.138+/-0.013 muM and selectivity index of 0.0103. The inhibition of resveratrol (MAO-A) and pterostilbene (MAO-B) was consistent with competitive time-independent mechanisms. Resveratrol 4'-glucoside was the only compound which inhibited MAO-A, but itself, resveratrol 3-glucoside, and pterostilbene 4'-glucoside failed to inhibit MAO-B. Additional studies are needed to establish the effects of these compounds on MAO-A and/or MAO-B in humans.
Contribution of the Alkylquinolone Quorum-Sensing System to the Interaction of Pseudomonas aeruginosa With Bronchial Epithelial Cells.[Pubmed:30619119]
Front Microbiol. 2018 Dec 18;9:3018.
Pseudomonas aeruginosa causes infections in patients with compromised epithelial barrier function. Multiple virulence factors produced by P. aeruginosa are controlled by quorum sensing (QS) via 2-alkyl-4(1H)-quinolone (AQ) signal molecules. Here, we investigated the impact of AQs on P. aeruginosa PAO1 infection of differentiated human bronchial epithelial cells (HBECs). The pqsA-E operon is responsible for the biosynthesis of AQs including the 2-alkyl-3-hydroxy-4-quinolones, 4-hydroxy-2-alkylquinolines, and 4-hydroxy-2-alkylquinoline N-oxides as exemplified by pseudomonas quinolone signal (PQS), 2-heptyl-4-Hydroxyquinoline (HHQ), and 2-heptyl-4-Hydroxyquinoline N-oxide (HQNO), respectively. PQS and HHQ both act as QS signal molecules while HQNO is a cytochrome inhibitor. PqsE contributes both to AQ biosynthesis and promotes virulence in a PQS-independent manner. Our results show that PQS, HHQ, and HQNO were produced during PAO1 infection of HBECs, but no differences in growth or cytotoxicity were apparent when PAO1 and an AQ-negative DeltapqsA mutant were compared. Both strains promoted synthesis of inflammatory cytokines TNF-alpha, interleukin (IL)-6, and IL-17C by HBECs, and the provision of exogenous PQS negatively impacted on this response without affecting bacterial growth. Expression of pqsE and the PQS-independent PqsE-regulated genes mexG and lecA was detected during HBEC infection. Levels were reduced in the DeltapqsA mutant, that is, in the absence of PQS, and increased by exogenous PQS. These results support an AQ-independent role for PqsE during initial infection of HBEC by P. aeruginosa and for PQS as an enhancer of PqsE and PqsE-controlled virulence determinants and as an immunomodulator.