Fenfangjine GCAS# 205533-81-9 |
Quality Control & MSDS
3D structure
Package In Stock
Number of papers citing our products
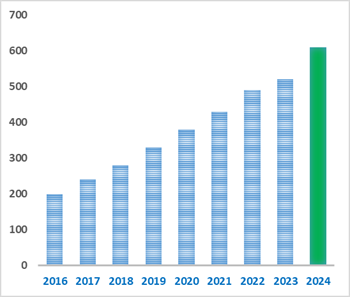
Cas No. | 205533-81-9 | SDF | Download SDF |
PubChem ID | 102004748 | Appearance | Powder |
Formula | C22H27NO8 | M.Wt | 433.5 |
Type of Compound | Alkaloids | Storage | Desiccate at -20°C |
Solubility | Soluble in Chloroform,Dichloromethane,Ethyl Acetate,DMSO,Acetone,etc. | ||
SMILES | CC(=O)OC1CC23CCNC(C2=C(C1OC(=O)C)OC)C(C4=C3C(=C(C=C4)OC)O)O | ||
Standard InChIKey | YVYLKUBETBPYFU-BQMSLCBESA-N | ||
Standard InChI | InChI=1S/C22H27NO8/c1-10(24)30-14-9-22-7-8-23-17(16(22)21(29-4)20(14)31-11(2)25)18(26)12-5-6-13(28-3)19(27)15(12)22/h5-6,14,17-18,20,23,26-27H,7-9H2,1-4H3/t14?,17-,18?,20?,22+/m1/s1 | ||
General tips | For obtaining a higher solubility , please warm the tube at 37 ℃ and shake it in the ultrasonic bath for a while.Stock solution can be stored below -20℃ for several months. We recommend that you prepare and use the solution on the same day. However, if the test schedule requires, the stock solutions can be prepared in advance, and the stock solution must be sealed and stored below -20℃. In general, the stock solution can be kept for several months. Before use, we recommend that you leave the vial at room temperature for at least an hour before opening it. |
||
About Packaging | 1. The packaging of the product may be reversed during transportation, cause the high purity compounds to adhere to the neck or cap of the vial.Take the vail out of its packaging and shake gently until the compounds fall to the bottom of the vial. 2. For liquid products, please centrifuge at 500xg to gather the liquid to the bottom of the vial. 3. Try to avoid loss or contamination during the experiment. |
||
Shipping Condition | Packaging according to customer requirements(5mg, 10mg, 20mg and more). Ship via FedEx, DHL, UPS, EMS or other couriers with RT, or blue ice upon request. |

Fenfangjine G Dilution Calculator

Fenfangjine G Molarity Calculator
1 mg | 5 mg | 10 mg | 20 mg | 25 mg | |
1 mM | 2.3068 mL | 11.534 mL | 23.0681 mL | 46.1361 mL | 57.6701 mL |
5 mM | 0.4614 mL | 2.3068 mL | 4.6136 mL | 9.2272 mL | 11.534 mL |
10 mM | 0.2307 mL | 1.1534 mL | 2.3068 mL | 4.6136 mL | 5.767 mL |
50 mM | 0.0461 mL | 0.2307 mL | 0.4614 mL | 0.9227 mL | 1.1534 mL |
100 mM | 0.0231 mL | 0.1153 mL | 0.2307 mL | 0.4614 mL | 0.5767 mL |
* Note: If you are in the process of experiment, it's necessary to make the dilution ratios of the samples. The dilution data above is only for reference. Normally, it's can get a better solubility within lower of Concentrations. |
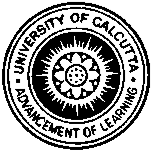
Calcutta University
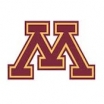
University of Minnesota
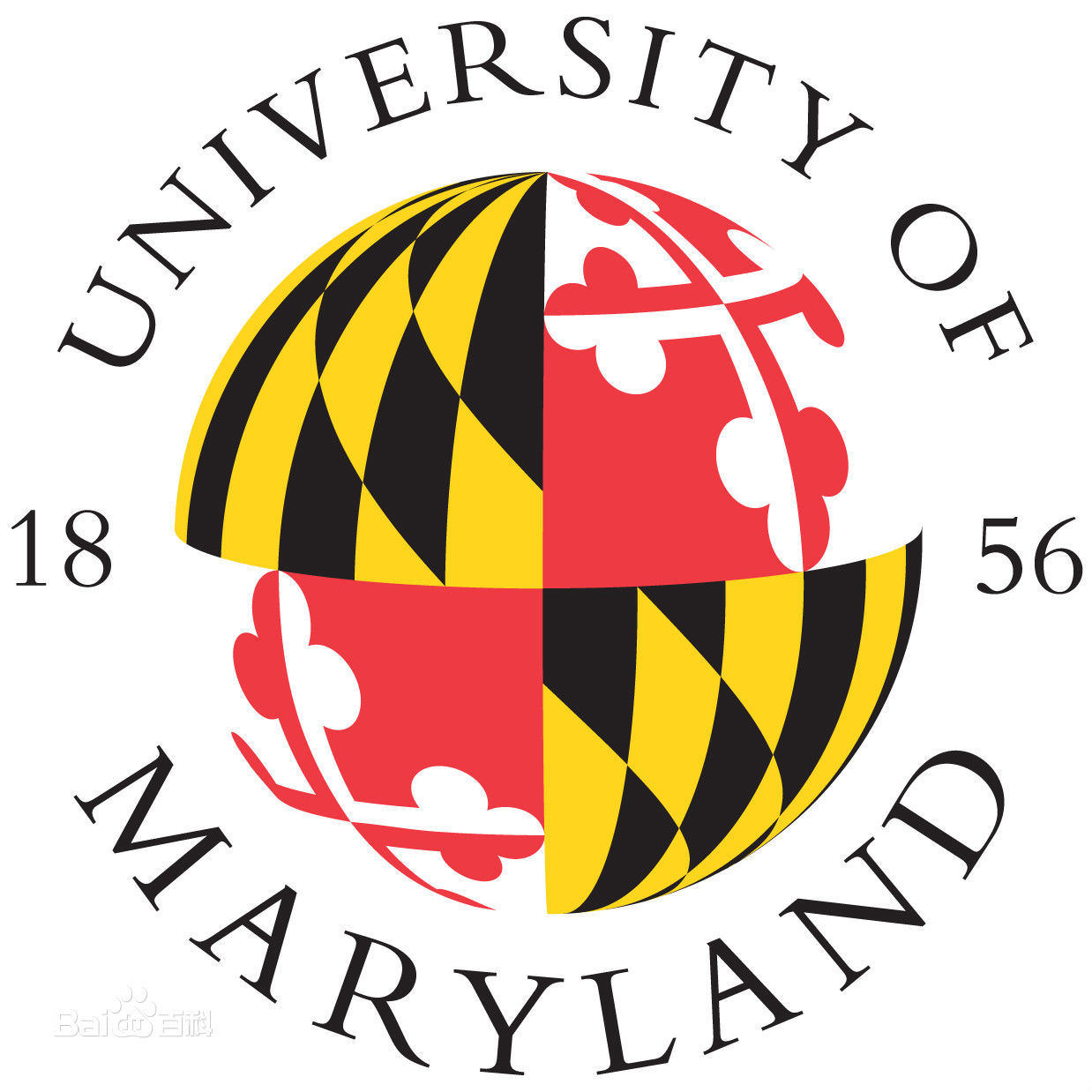
University of Maryland School of Medicine
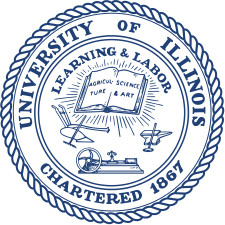
University of Illinois at Chicago
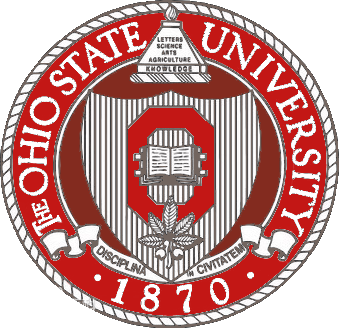
The Ohio State University
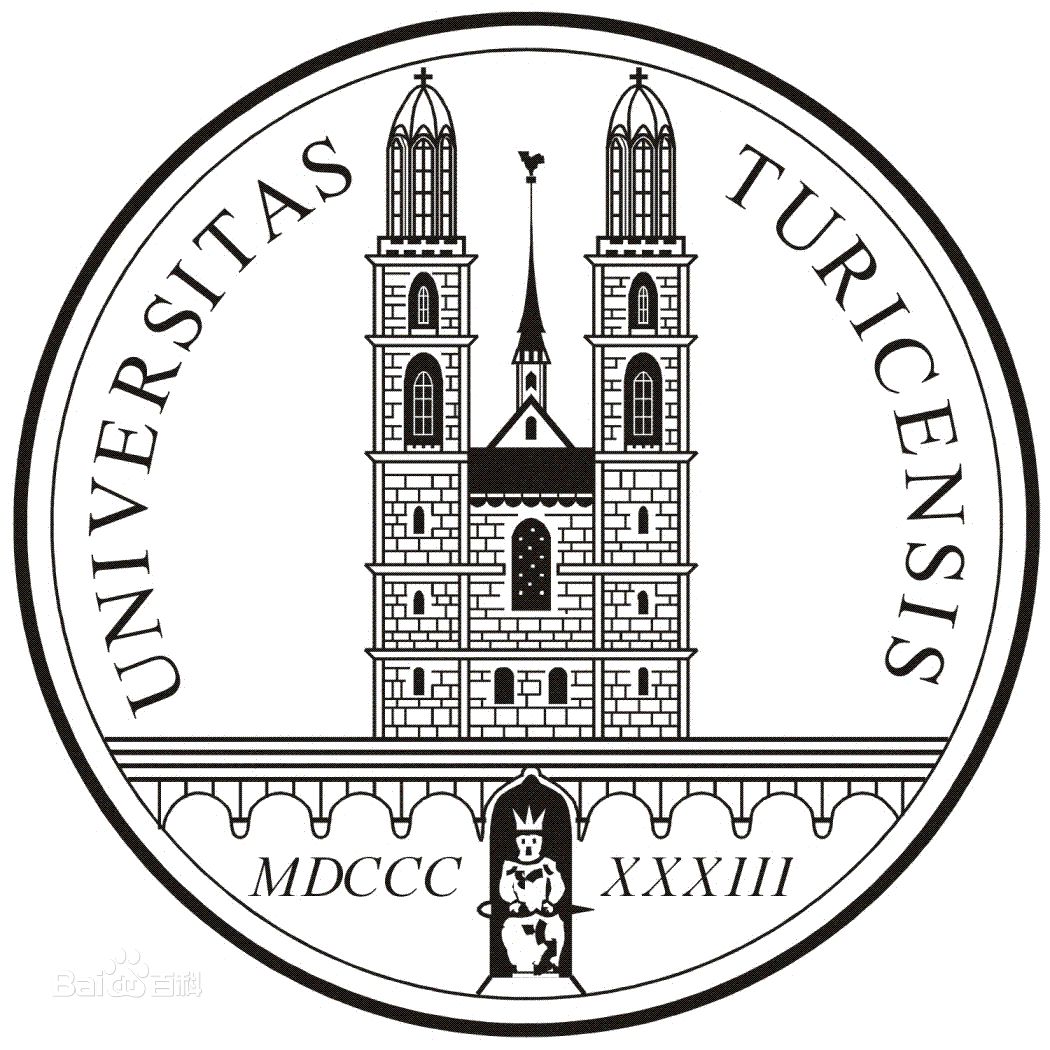
University of Zurich
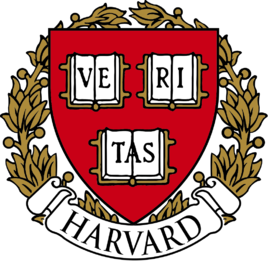
Harvard University
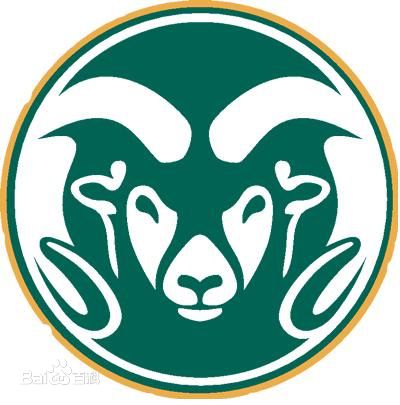
Colorado State University
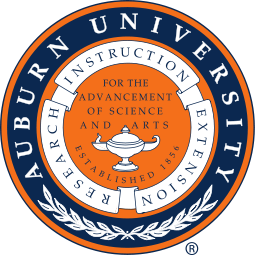
Auburn University
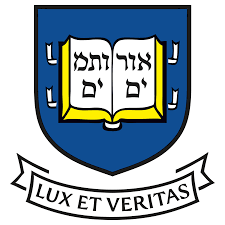
Yale University
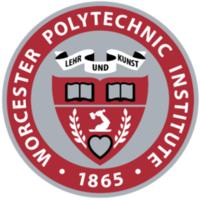
Worcester Polytechnic Institute
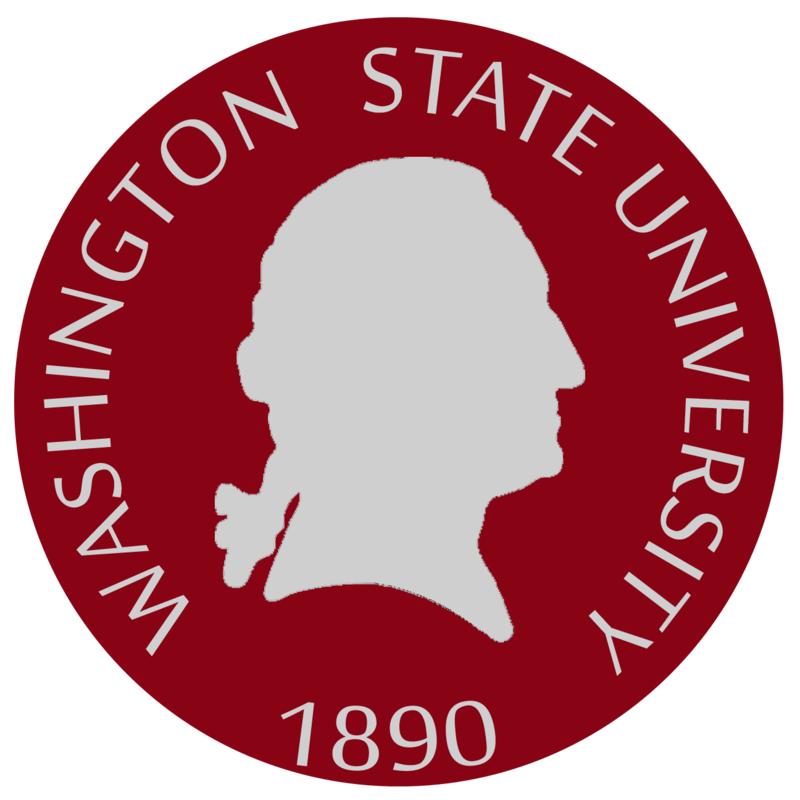
Washington State University
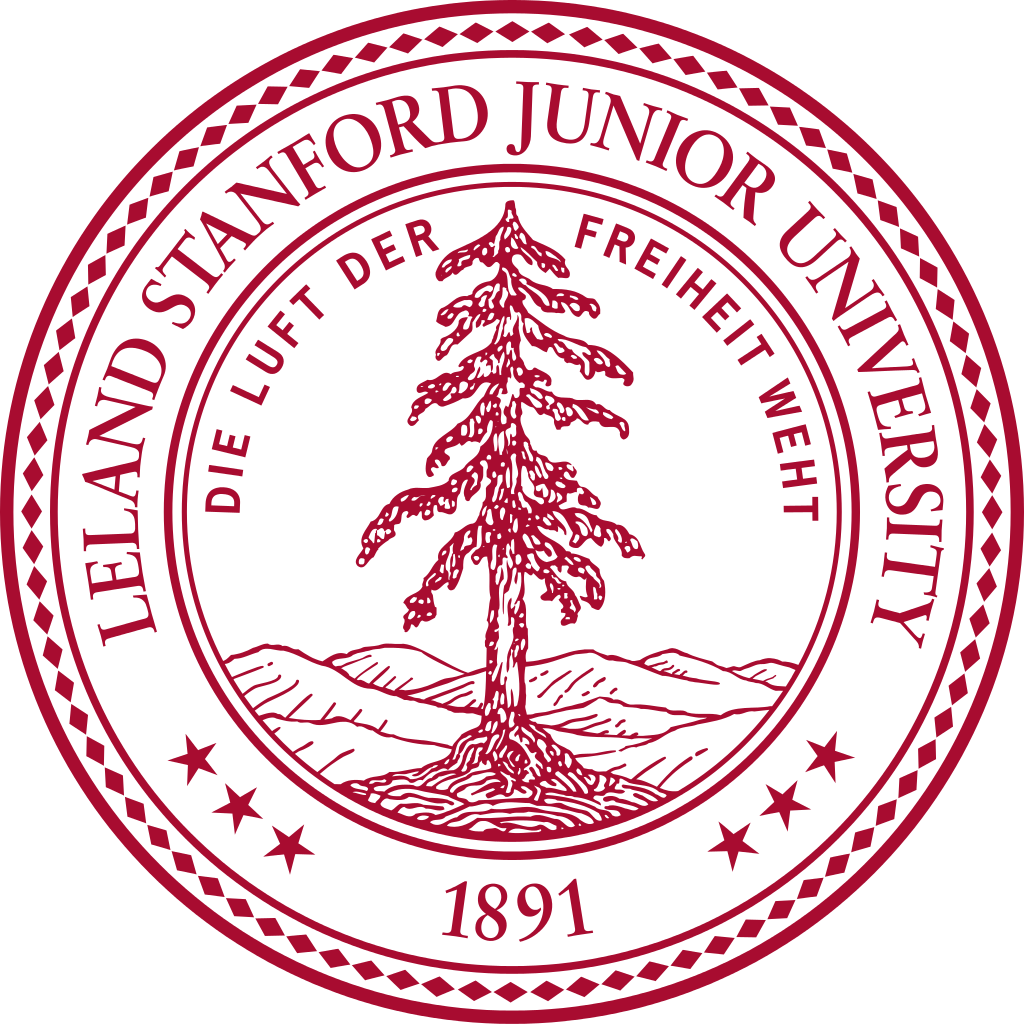
Stanford University
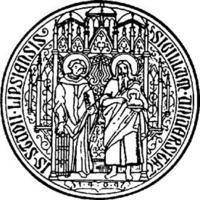
University of Leipzig
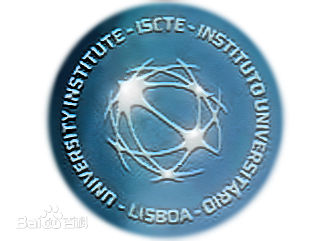
Universidade da Beira Interior
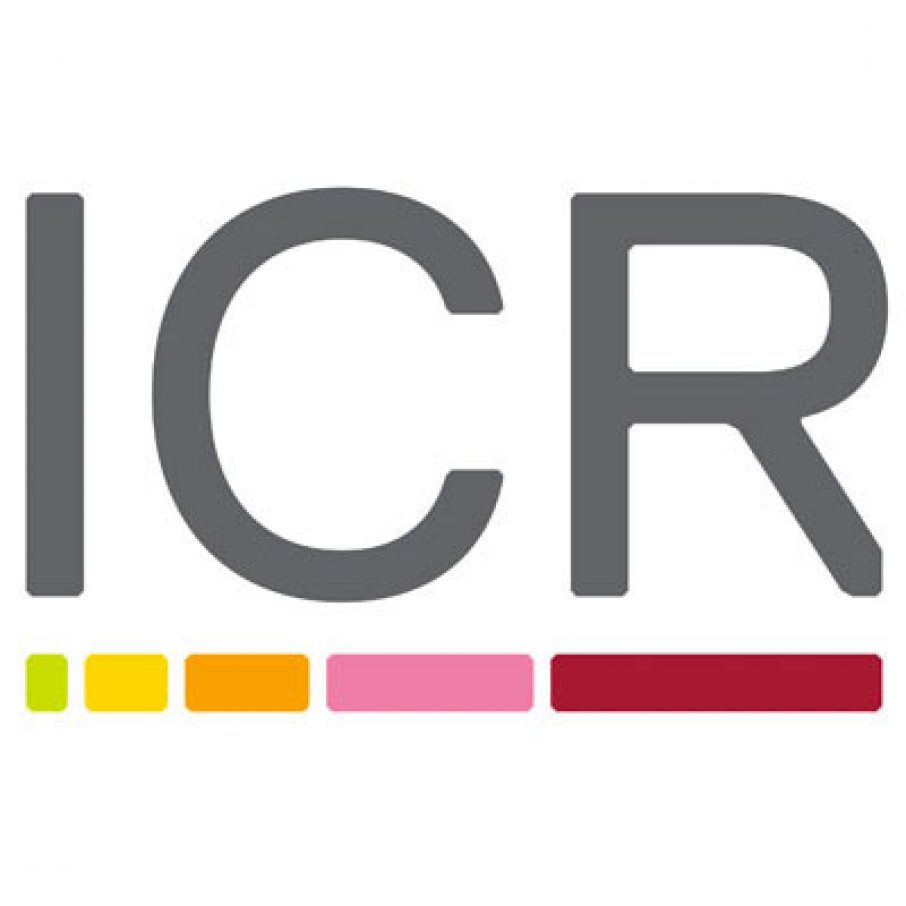
The Institute of Cancer Research
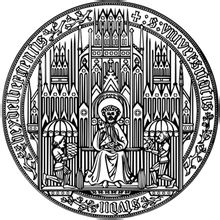
Heidelberg University
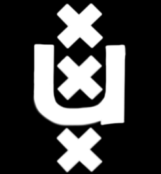
University of Amsterdam
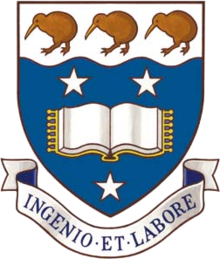
University of Auckland
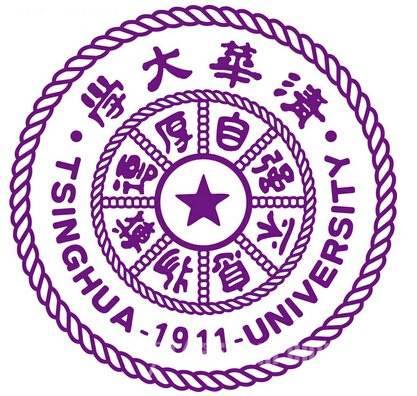
TsingHua University
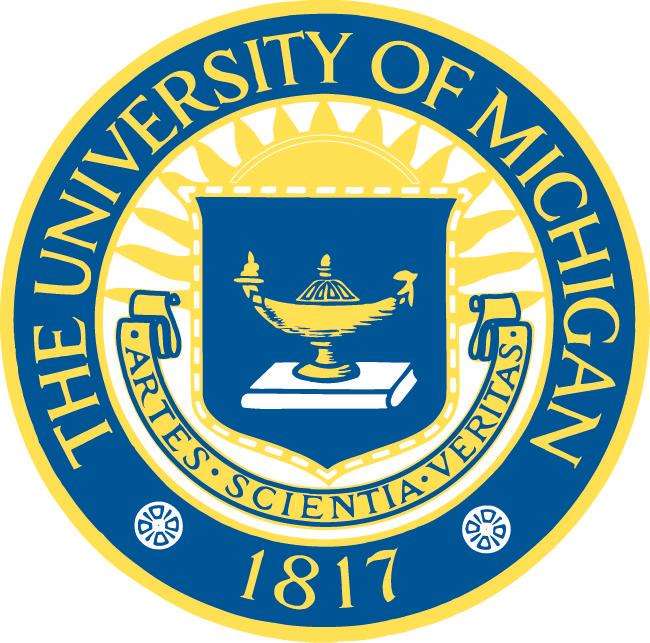
The University of Michigan
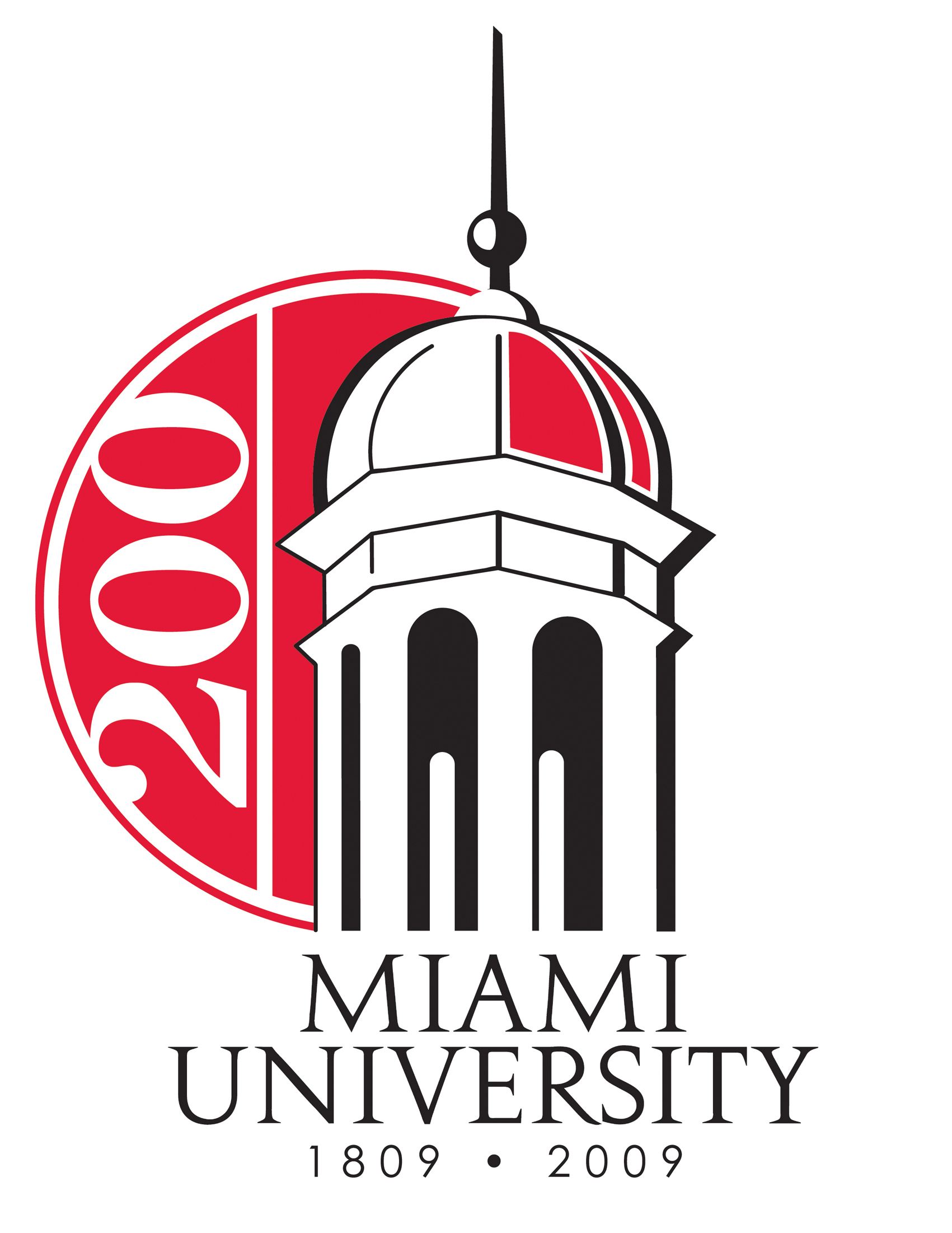
Miami University
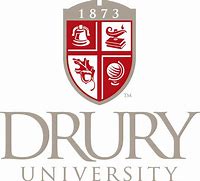
DRURY University
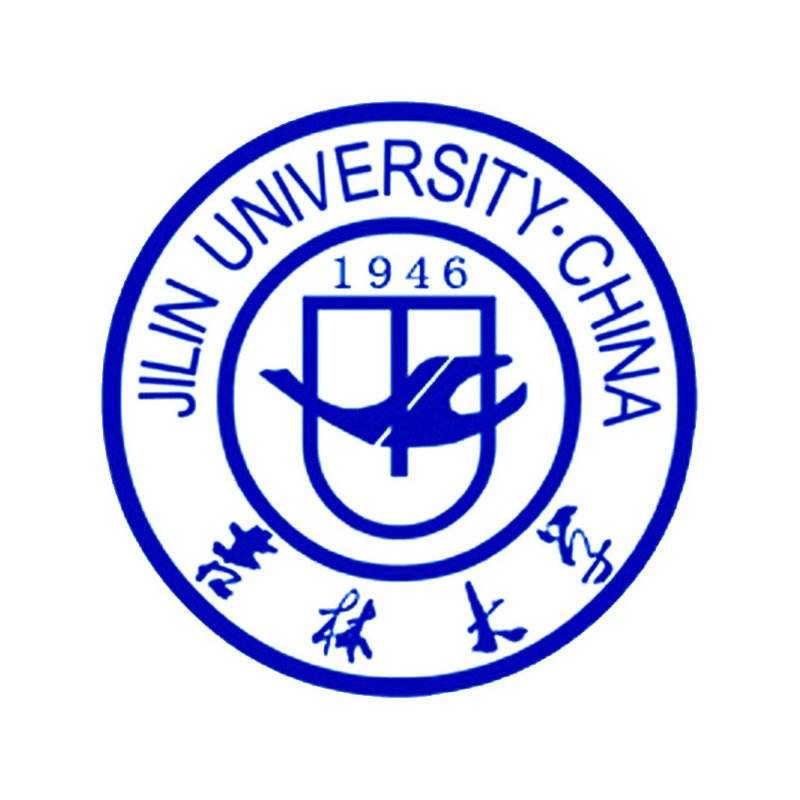
Jilin University
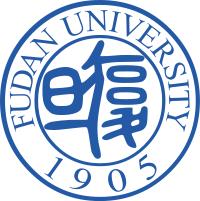
Fudan University
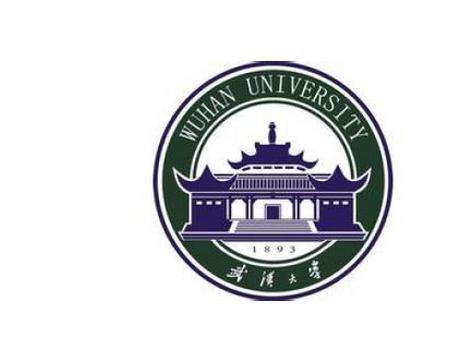
Wuhan University
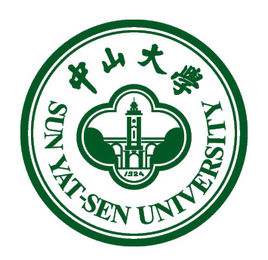
Sun Yat-sen University
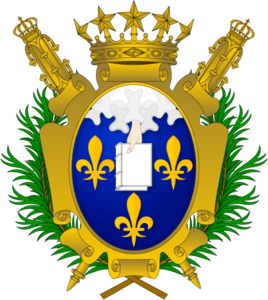
Universite de Paris
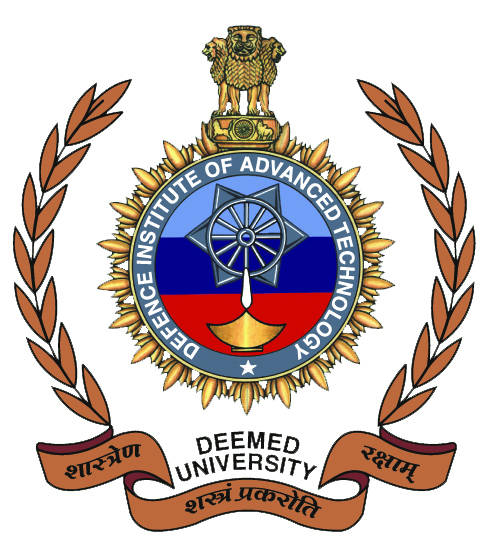
Deemed University
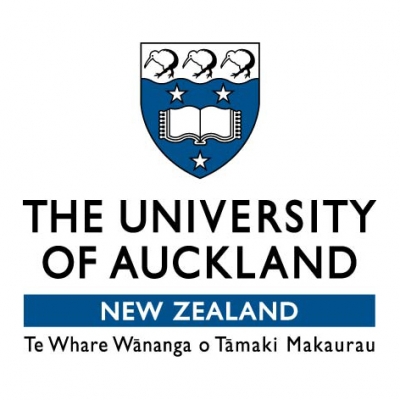
Auckland University
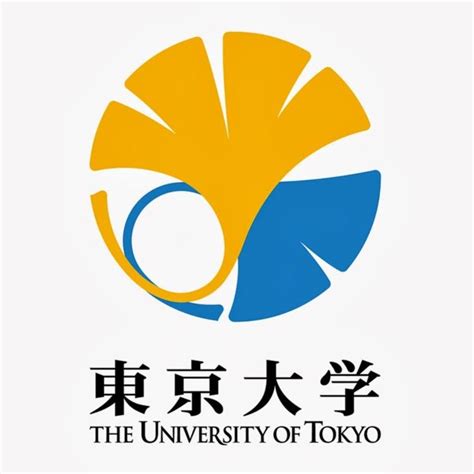
The University of Tokyo
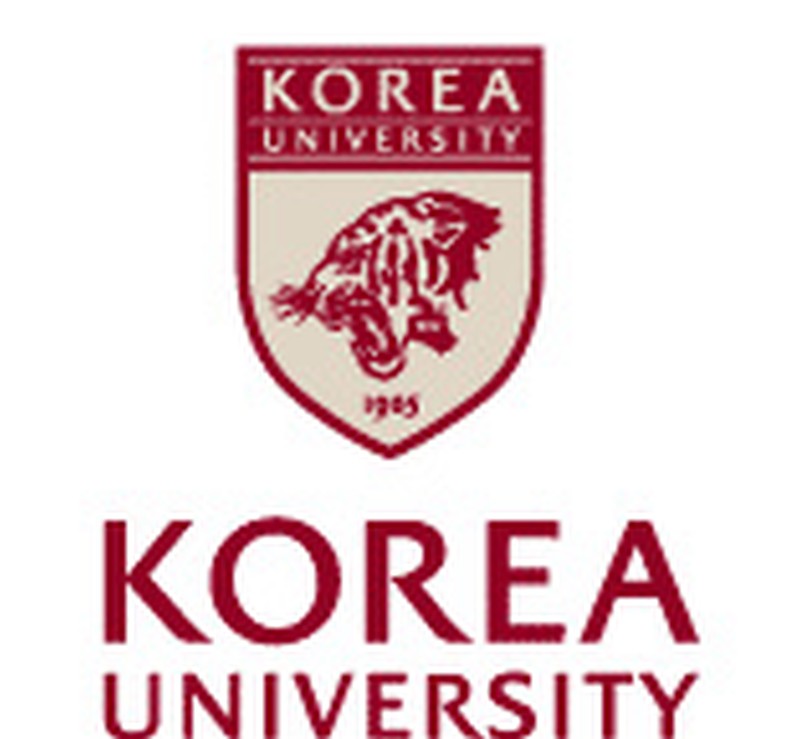
Korea University
- Fmoc-D-Phe(4-I)-OH
Catalog No.:BCC3262
CAS No.:205526-29-0
- Fmoc-D-Phe(3-Cl)-OH
Catalog No.:BCC3170
CAS No.:205526-23-4
- Rauvovertine A
Catalog No.:BCN7728
CAS No.:2055073-75-9
- Rauvovertine C
Catalog No.:BCN7727
CAS No.:2055073-74-8
- Rauvovertine B
Catalog No.:BCN7726
CAS No.:2055073-72-6
- Paederoside
Catalog No.:BCN3437
CAS No.:20547-45-9
- Taxezopidine G
Catalog No.:BCN6947
CAS No.:205440-22-8
- BU 224 hydrochloride
Catalog No.:BCC6765
CAS No.:205437-64-5
- Pinocembrin 7-O-(3'-galloyl-4',6'-(S)-hexahydroxydiphenoyl)-beta-D-glucose
Catalog No.:BCN6769
CAS No.:205370-59-8
- Amifostine
Catalog No.:BCC5232
CAS No.:20537-88-6
- DL-TBOA
Catalog No.:BCC5735
CAS No.:205309-81-5
- Isonardoperoxide
Catalog No.:BCN7628
CAS No.:205248-65-3
- 2'',4''-Di-O-(Z-p-coumaroyl)afzelin
Catalog No.:BCN4676
CAS No.:205534-17-4
- Parthenolide
Catalog No.:BCN4896
CAS No.:20554-84-1
- Faradiol
Catalog No.:BCN3795
CAS No.:20554-95-4
- Pterocarpadiol B
Catalog No.:BCN7757
CAS No.:2055882-20-5
- Pterocarpadiol A
Catalog No.:BCN7758
CAS No.:2055882-21-6
- Pterocarpadiol C
Catalog No.:BCN7759
CAS No.:2055882-22-7
- Pterocarpadiol D
Catalog No.:BCN7760
CAS No.:2055882-23-8
- Oxibendazole
Catalog No.:BCC4818
CAS No.:20559-55-1
- alpha-Solanine
Catalog No.:BCN2701
CAS No.:20562-02-1
- alpha-Chaconine
Catalog No.:BCN2162
CAS No.:20562-03-2
- Orexin A (human, rat, mouse)
Catalog No.:BCC5764
CAS No.:205640-90-0
- Orexin B (human)
Catalog No.:BCC5765
CAS No.:205640-91-1
The effects of DNA supercoiling on G-quadruplex formation.[Pubmed:29036619]
Nucleic Acids Res. 2017 Dec 1;45(21):12069-12079.
Guanine-rich DNAs can fold into four-stranded structures that contain stacks of G-quartets. Bioinformatics studies have revealed that G-rich sequences with the potential to adopt these structures are unevenly distributed throughout genomes, and are especially found in gene promoter regions. With the exception of the single-stranded telomeric DNA, all genomic G-rich sequences will always be present along with their C-rich complements, and quadruplex formation will be in competition with the corresponding Watson-Crick duplex. Quadruplex formation must therefore first require local dissociation (melting) of the duplex strands. Since negative supercoiling is known to facilitate the formation of alternative DNA structures, we have investigated G-quadruplex formation within negatively supercoiled DNA plasmids. Plasmids containing multiple copies of (G3T)n and (G3T4)n repeats, were probed with dimethylsulphate, potassium permanganate and S1 nuclease. While dimethylsulphate footprinting revealed some evidence for G-quadruplex formation in (G3T)n sequences, this was not affected by supercoiling, and permanganate failed to detect exposed thymines in the loop regions. (G3T4)n sequences were not protected from DMS and showed no reaction with permanganate. Similarly, both S1 nuclease and 2D gel electrophoresis of DNA topoisomers did not detect any supercoil-dependent structural transitions. These results suggest that negative supercoiling alone is not sufficient to drive G-quadruplex formation.
Motif independent identification of potential RNA G-quadruplexes by G4RNA screener.[Pubmed:29036425]
Bioinformatics. 2017 Nov 15;33(22):3532-3537.
Motivation: G-quadruplex structures in RNA molecules are known to have regulatory impacts in cells but are difficult to locate in the genome. The minimal requirements for G-quadruplex folding in RNA (G>/=3N1-7 G>/=3N1-7 G>/=3N1-7 G>/=3) is being challenged by observations made on specific examples in recent years. The definition of potential G-quadruplex sequences has major repercussions on the observation of the structure since it introduces a bias. The canonical motif only describes a sub-population of the reported G-quadruplexes. To address these issues, we propose an RNA G-quadruplex prediction strategy that does not rely on a motif definition. Results: We trained an artificial neural network with sequences of experimentally validated G-quadruplexes from the G4RNA database encoded using an abstract definition of their sequence. This artificial neural network, G4NN, evaluates the similarity of a given sequence to known G-quadruplexes and reports it as a score. G4NN has a predictive power comparable to the reported G richness and G/C skewness evaluations that are the current state-of-the-art for the identification of potential RNA G-quadruplexes. We combined these approaches in the G4RNA screener, a program designed to manage and evaluate the sequences to identify potential G-quadruplexes. Availability and implementation: G4RNA screener is available for download at http://gitlabscottgroup.med.usherbrooke.ca/J-Michel/g4rna_screener. Contact: jean-michel.garant@usherbrooke.ca or jean-pierre.perreault@usherbrooke.ca or michelle.scott@usherbrooke.ca. Supplementary information: Supplementary data are available at Bioinformatics online.
2'-Fluoroarabinonucleic acid modification traps G-quadruplex and i-motif structures in human telomeric DNA.[Pubmed:29036537]
Nucleic Acids Res. 2017 Nov 16;45(20):11535-11546.
Human telomeres and promoter regions of genes fulfill a significant role in cellular aging and cancer. These regions comprise of guanine and cytosine-rich repeats, which under certain conditions can fold into G-quadruplex (G4) and i-motif structures, respectively. Herein, we use UV, circular dichroism and NMR spectroscopy to study several human telomeric sequences and demonstrate that G4/i-motif-duplex interconversion kinetics are slowed down dramatically by 2'-beta-fluorination and the presence of G4/i-motif-duplex junctions. NMR-monitored kinetic experiments on 1:1 mixtures of native and modified C- and G-rich human telomeric sequences reveal that strand hybridization kinetics are controlled by G4 or i-motif unfolding. Furthermore, we provide NMR evidence for the formation of a hybrid complex containing G4 and i-motif structures proximal to a duplex DNA segment at neutral pH. While the presence of i-motif and G4 folds may be mutually exclusive in promoter genome sequences, our results suggest that they may co-exist transiently as intermediates in telomeric sequences.
Glioma CpG island methylator phenotype (G-CIMP): biological and clinical implications.[Pubmed:29036500]
Neuro Oncol. 2018 Apr 9;20(5):608-620.
Gliomas are a heterogeneous group of brain tumors with distinct biological and clinical properties. Despite advances in surgical techniques and clinical regimens, treatment of high-grade glioma remains challenging and carries dismal rates of therapeutic success and overall survival. Challenges include the molecular complexity of gliomas, as well as inconsistencies in histopathological grading, resulting in an inaccurate prediction of disease progression and failure in the use of standard therapy. The updated 2016 World Health Organization (WHO) classification of tumors of the central nervous system reflects a refinement of tumor diagnostics by integrating the genotypic and phenotypic features, thereby narrowing the defined subgroups. The new classification recommends molecular diagnosis of isocitrate dehydrogenase (IDH) mutational status in gliomas. IDH-mutant gliomas manifest the cytosine-phosphate-guanine (CpG) island methylator phenotype (G-CIMP). Notably, the recent identification of clinically relevant subsets of G-CIMP tumors (G-CIMP-high and G-CIMP-low) provides a further refinement in glioma classification that is independent of grade and histology. This scheme may be useful for predicting patient outcome and may be translated into effective therapeutic strategies tailored to each patient. In this review, we highlight the evolution of our understanding of the G-CIMP subsets and how recent advances in characterizing the genome and epigenome of gliomas may influence future basic and translational research.
When Heterotrimeric G Proteins Are Not Activated by G Protein-Coupled Receptors: Structural Insights and Evolutionary Conservation.[Pubmed:29035513]
Biochemistry. 2018 Jan 23;57(3):255-257.
Heterotrimeric G proteins are signal-transducing switches conserved across eukaryotes. In humans, they work as critical mediators of intercellular communication in the context of virtually any physiological process. While G protein regulation by G protein-coupled receptors (GPCRs) is well-established and has received much attention, it has become recently evident that heterotrimeric G proteins can also be activated by cytoplasmic proteins. However, this alternative mechanism of G protein regulation remains far less studied than GPCR-mediated signaling. This Viewpoint focuses on recent advances in the characterization of a group of nonreceptor proteins that contain a sequence dubbed the "Galpha-binding and -activating (GBA) motif". So far, four proteins present in mammals [GIV (also known as Girdin), DAPLE, CALNUC, and NUCB2] and one protein in Caenorhabditis elegans (GBAS-1) have been described as possessing a functional GBA motif. The GBA motif confers guanine nucleotide exchange factor activity on Galphai subunits in vitro and activates G protein signaling in cells. The importance of this mechanism of signal transduction is highlighted by the fact that its dysregulation underlies human diseases, such as cancer, which has made the proteins attractive new candidates for therapeutic intervention. Here we discuss recent discoveries on the structural basis of GBA-mediated activation of G proteins and its evolutionary conservation and compare them with the better-studied mechanism mediated by GPCRs.