PantolactoneCAS# 599-04-2 |
Quality Control & MSDS
3D structure
Package In Stock
Number of papers citing our products
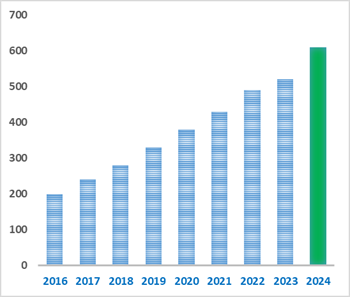
Cas No. | 599-04-2 | SDF | Download SDF |
PubChem ID | 439368.0 | Appearance | Powder |
Formula | C6H10O3 | M.Wt | 130.14 |
Type of Compound | N/A | Storage | Desiccate at -20°C |
Solubility | Soluble in Chloroform,Dichloromethane,Ethyl Acetate,DMSO,Acetone,etc. | ||
Chemical Name | (3R)-3-hydroxy-4,4-dimethyloxolan-2-one | ||
SMILES | CC1(COC(=O)C1O)C | ||
Standard InChIKey | SERHXTVXHNVDKA-BYPYZUCNSA-N | ||
Standard InChI | InChI=1S/C6H10O3/c1-6(2)3-9-5(8)4(6)7/h4,7H,3H2,1-2H3/t4-/m0/s1 | ||
General tips | For obtaining a higher solubility , please warm the tube at 37 ℃ and shake it in the ultrasonic bath for a while.Stock solution can be stored below -20℃ for several months. We recommend that you prepare and use the solution on the same day. However, if the test schedule requires, the stock solutions can be prepared in advance, and the stock solution must be sealed and stored below -20℃. In general, the stock solution can be kept for several months. Before use, we recommend that you leave the vial at room temperature for at least an hour before opening it. |
||
About Packaging | 1. The packaging of the product may be reversed during transportation, cause the high purity compounds to adhere to the neck or cap of the vial.Take the vail out of its packaging and shake gently until the compounds fall to the bottom of the vial. 2. For liquid products, please centrifuge at 500xg to gather the liquid to the bottom of the vial. 3. Try to avoid loss or contamination during the experiment. |
||
Shipping Condition | Packaging according to customer requirements(5mg, 10mg, 20mg and more). Ship via FedEx, DHL, UPS, EMS or other couriers with RT, or blue ice upon request. |

Pantolactone Dilution Calculator

Pantolactone Molarity Calculator
1 mg | 5 mg | 10 mg | 20 mg | 25 mg | |
1 mM | 7.684 mL | 38.4202 mL | 76.8403 mL | 153.6807 mL | 192.1008 mL |
5 mM | 1.5368 mL | 7.684 mL | 15.3681 mL | 30.7361 mL | 38.4202 mL |
10 mM | 0.7684 mL | 3.842 mL | 7.684 mL | 15.3681 mL | 19.2101 mL |
50 mM | 0.1537 mL | 0.7684 mL | 1.5368 mL | 3.0736 mL | 3.842 mL |
100 mM | 0.0768 mL | 0.3842 mL | 0.7684 mL | 1.5368 mL | 1.921 mL |
* Note: If you are in the process of experiment, it's necessary to make the dilution ratios of the samples. The dilution data above is only for reference. Normally, it's can get a better solubility within lower of Concentrations. |
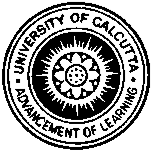
Calcutta University
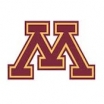
University of Minnesota
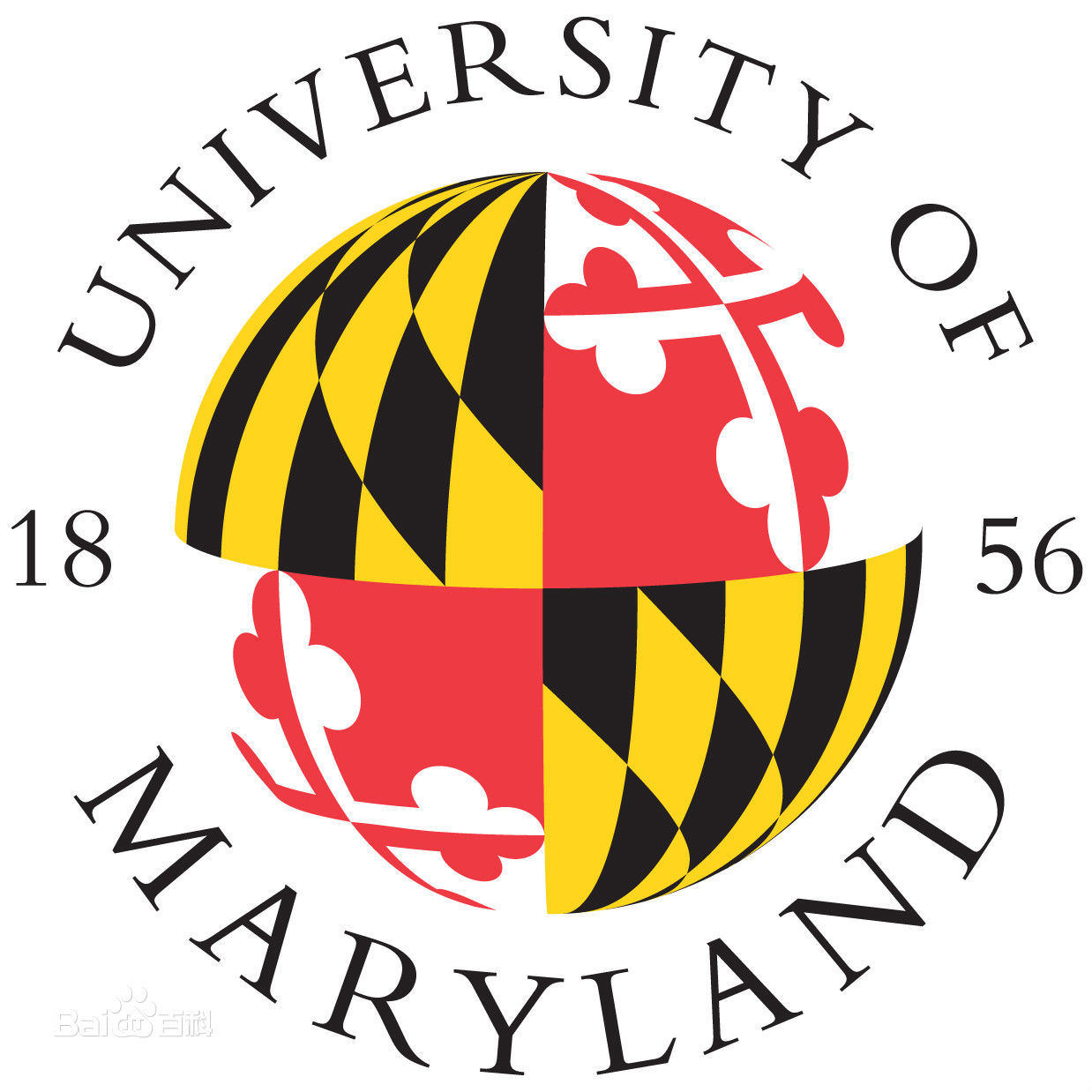
University of Maryland School of Medicine
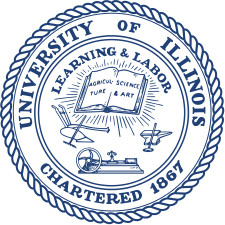
University of Illinois at Chicago
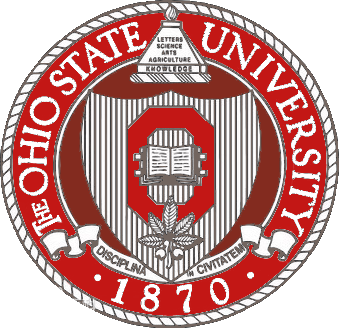
The Ohio State University
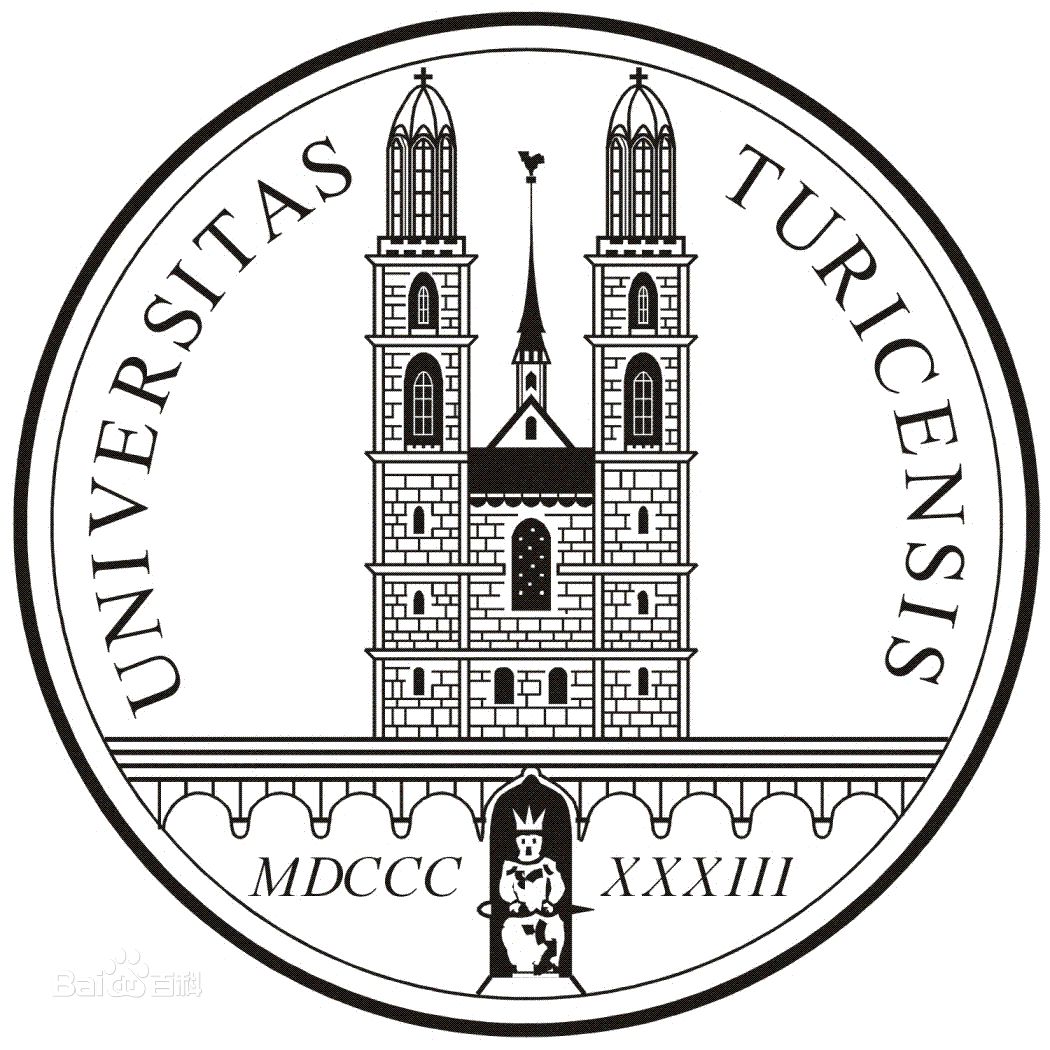
University of Zurich
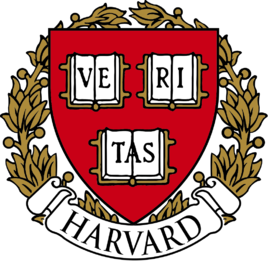
Harvard University
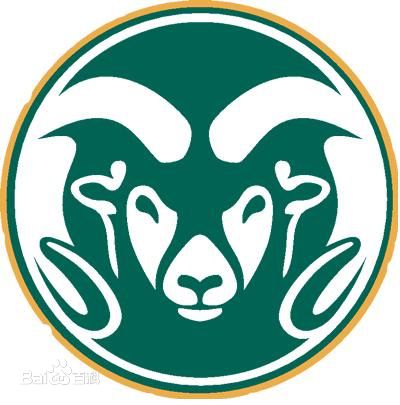
Colorado State University
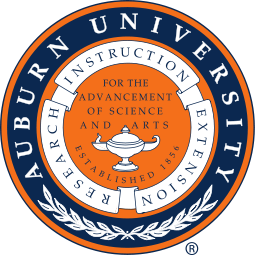
Auburn University
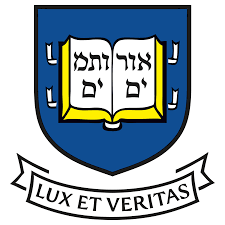
Yale University
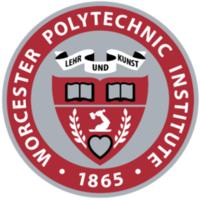
Worcester Polytechnic Institute
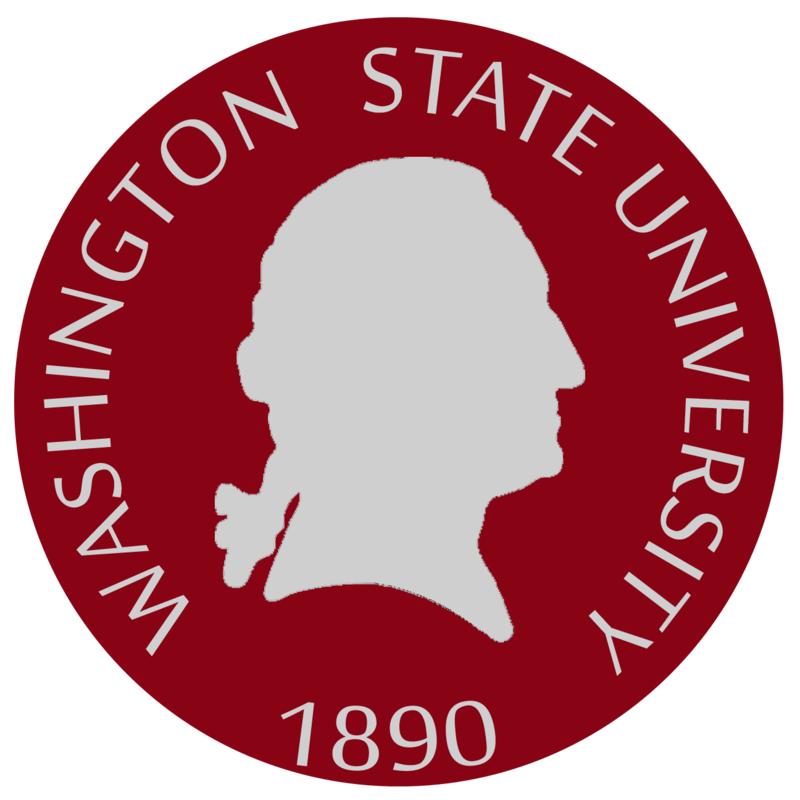
Washington State University
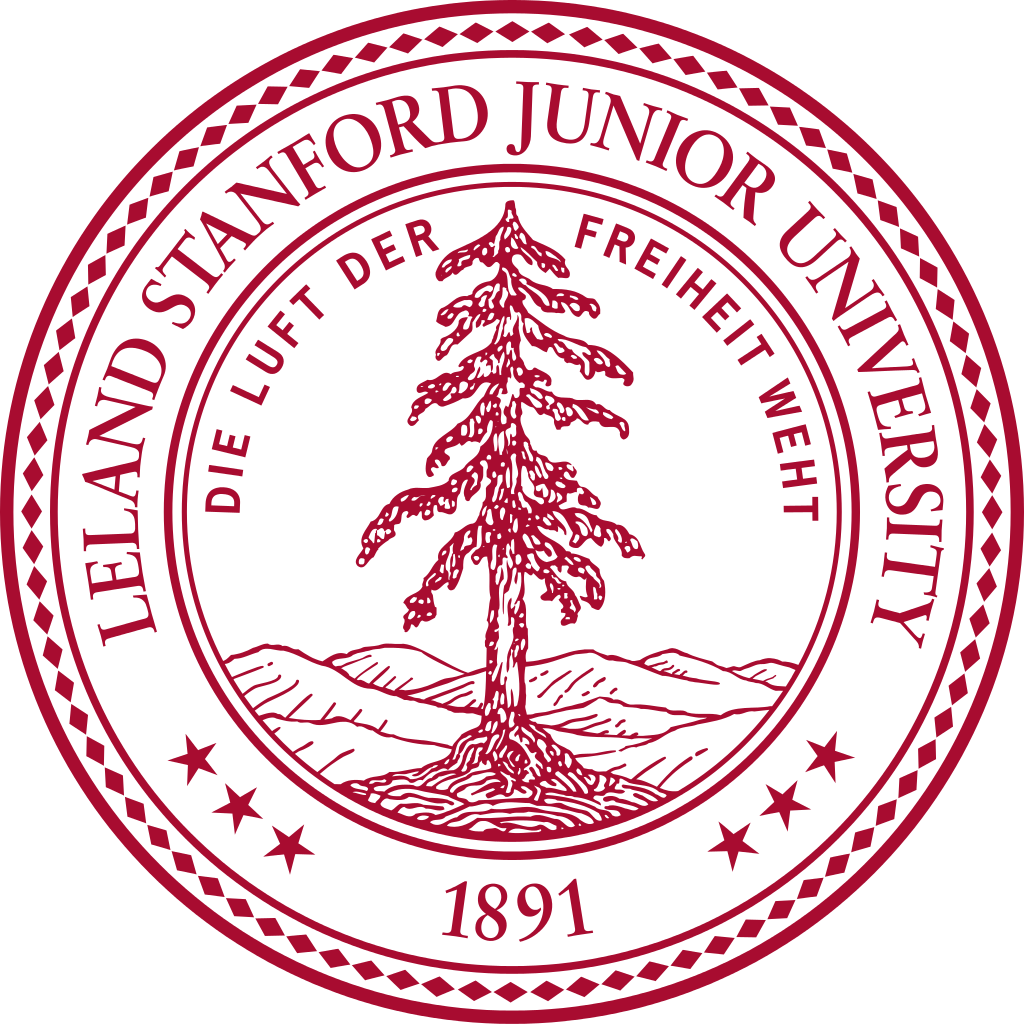
Stanford University
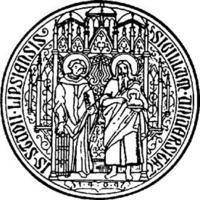
University of Leipzig
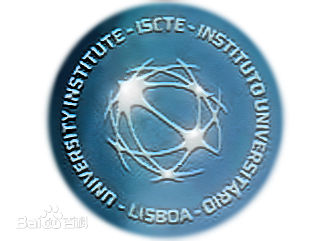
Universidade da Beira Interior
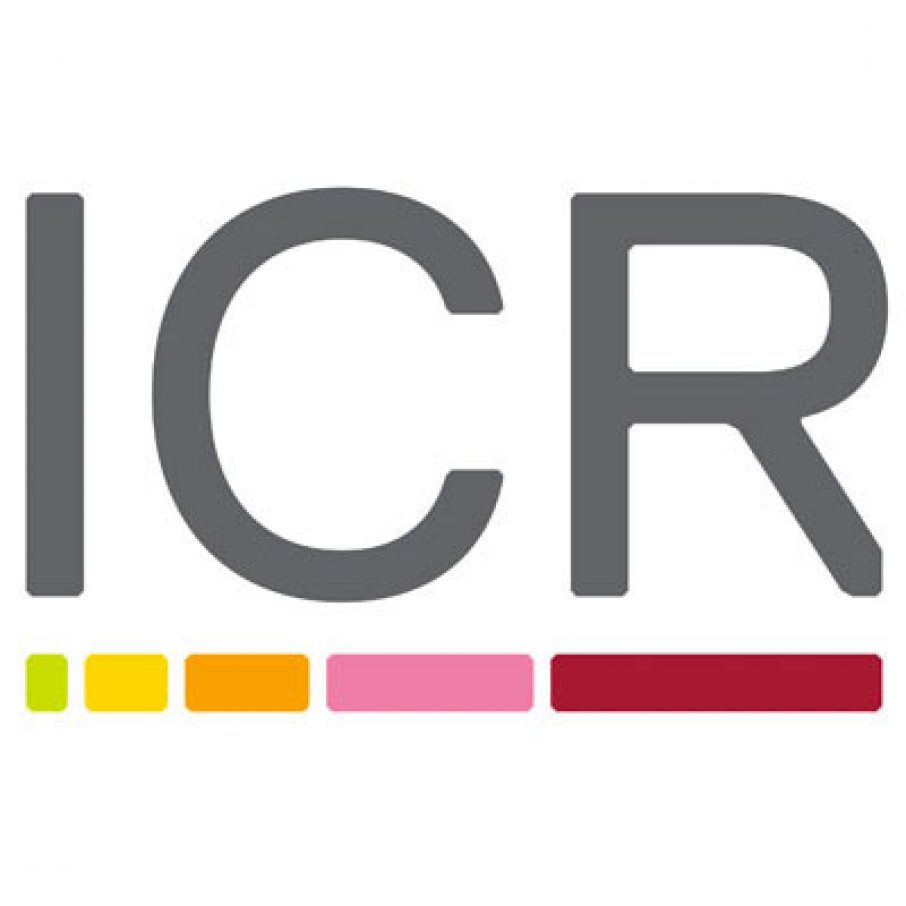
The Institute of Cancer Research
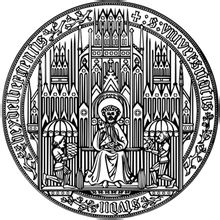
Heidelberg University
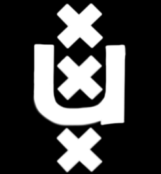
University of Amsterdam
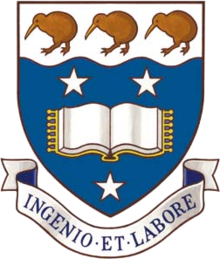
University of Auckland
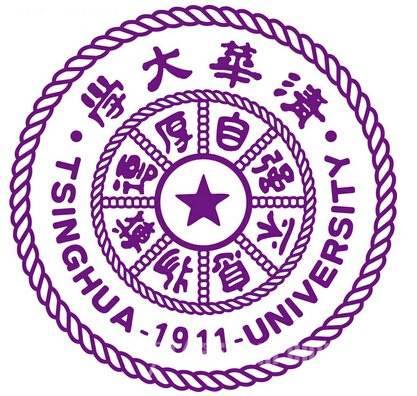
TsingHua University
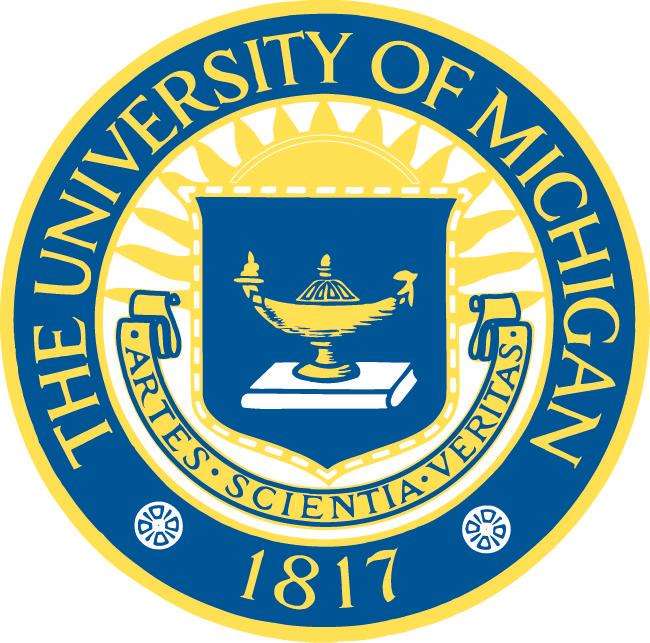
The University of Michigan
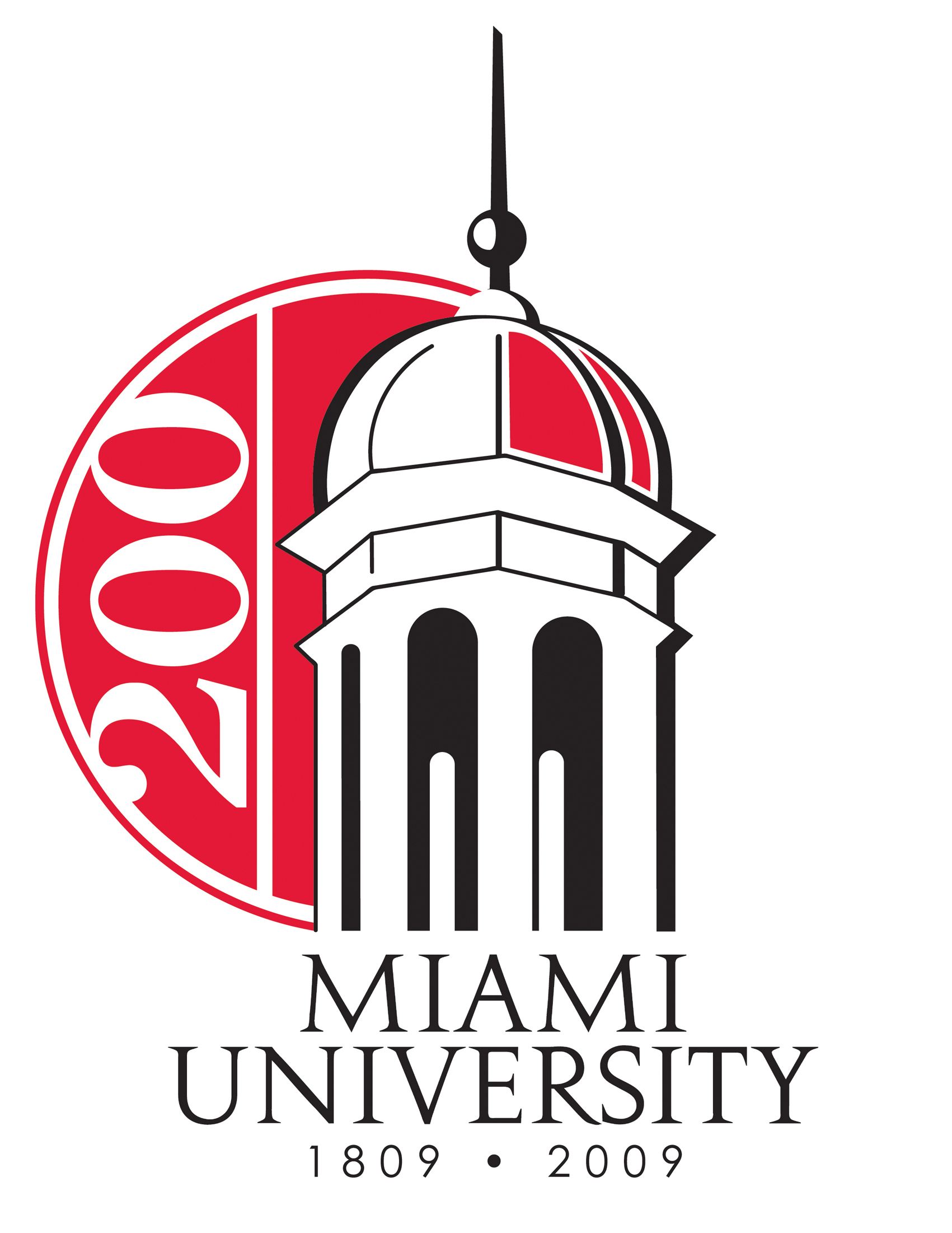
Miami University
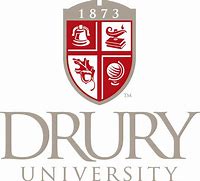
DRURY University
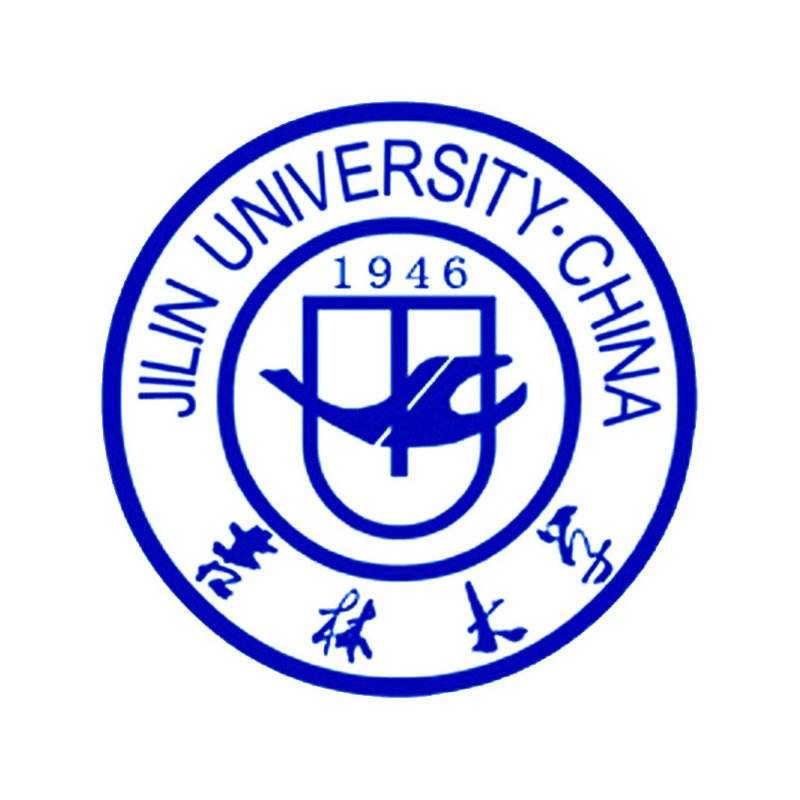
Jilin University
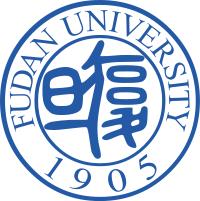
Fudan University
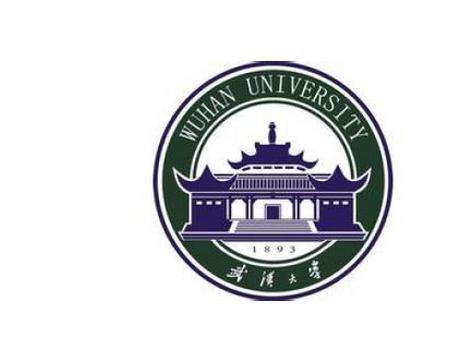
Wuhan University
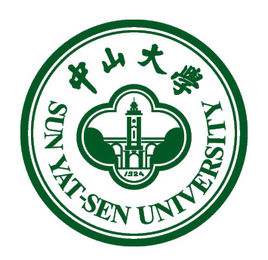
Sun Yat-sen University
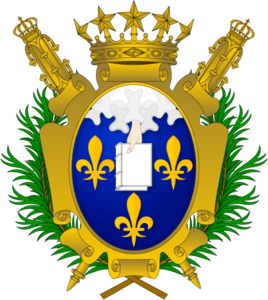
Universite de Paris
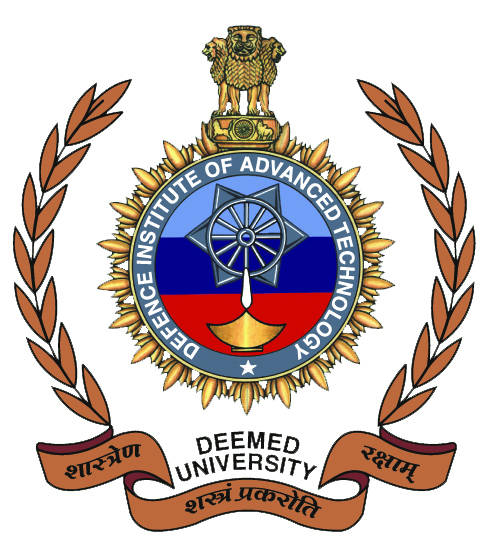
Deemed University
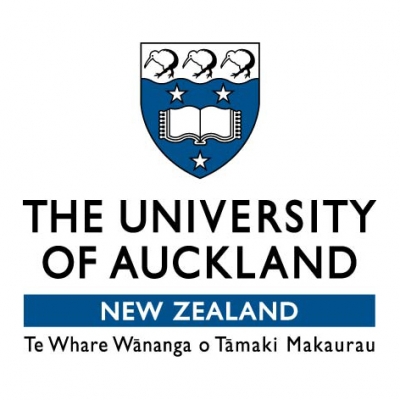
Auckland University
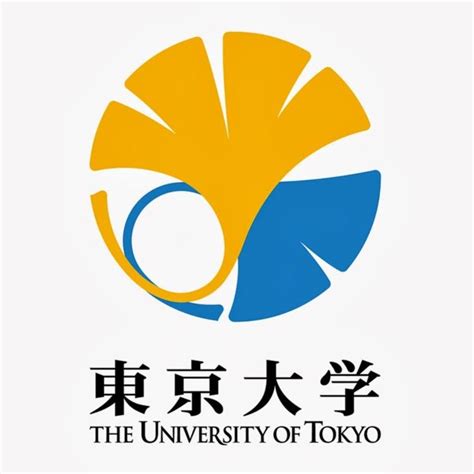
The University of Tokyo
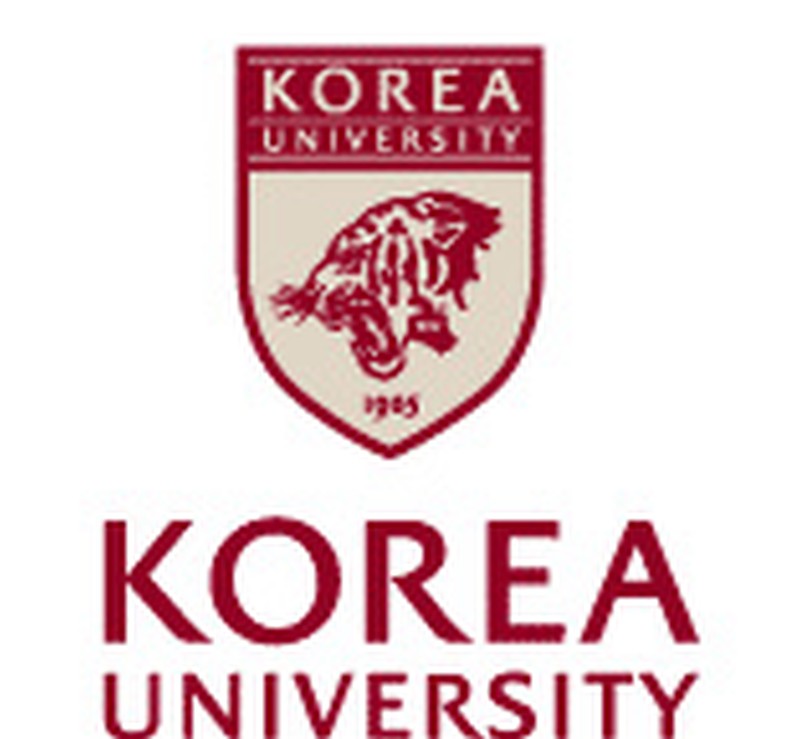
Korea University
- cis-Myrislignan
Catalog No.:BCX1556
CAS No.:52190-21-3
- Docosahexaenoic acid
Catalog No.:BCX1555
CAS No.:6217-54-5
- Delavinone
Catalog No.:BCX1554
CAS No.:96997-98-7
- Yubeinine
Catalog No.:BCX1553
CAS No.:157478-01-8
- Ebeiedinone
Catalog No.:BCX1552
CAS No.:25650-68-4
- Sibiricaxanthone A
Catalog No.:BCX1551
CAS No.:241125-76-8
- Dihydrolapachenole
Catalog No.:BCX1550
CAS No.:20213-26-7
- Lactiflorin
Catalog No.:BCX1549
CAS No.:1361049-59-3
- Rotundifuran
Catalog No.:BCX1548
CAS No.:50656-65-0
- Ursonic acid methyl ester
Catalog No.:BCX1547
CAS No.:989-72-0
- 8-Methyl Chrysophanol
Catalog No.:BCX1546
CAS No.:3300-25-2
- 7α-Hydroxycholesterol
Catalog No.:BCX1545
CAS No.:566-26-7
- 5-Methyl-5H-furan-2-one
Catalog No.:BCX1558
CAS No.:591-11-7
- Lutein laurate-myristate
Catalog No.:BCX1559
CAS No.:117116-36-6
- Lutein monostearate
Catalog No.:BCX1560
CAS No.:115156-87-1
- Lutein monopalmitate
Catalog No.:BCX1561
CAS No.:115182-29-1
- Lutein palmitate stearate
Catalog No.:BCX1562
CAS No.:79313-82-9
- Lutein monomyristate
Catalog No.:BCX1563
CAS No.:56842-49-0
- Lutein dimyristate
Catalog No.:BCX1564
CAS No.:86853-02-3
- Lutein myristate palmitate
Catalog No.:BCX1565
CAS No.:104784-49-8
- Lutein distearate
Catalog No.:BCX1566
CAS No.:86852-15-5
- Pinocembrin 7-O-[4'',6''-hexahydroxydiphenoyl]-β-D-glucoside
Catalog No.:BCX1567
CAS No.:205370-58-7
- 18α-Glycyrrhetinic acid Monoglucuronide
Catalog No.:BCX1568
CAS No.:400870-85-1
- α-Sanshool
Catalog No.:BCX1569
CAS No.:504-97-2
Enhancing the Catalytic Efficiency of D-lactonohydrolase through the Synergy of Tunnel Engineering, Evolutionary Analysis, and Force-Field Calculations.[Pubmed:38217521]
Chemistry. 2024 Mar 15;30(16):e202304164.
Computational design advances enzyme evolution and their use in biocatalysis in a faster and more efficient manner. In this study, a synergistic approach integrating tunnel engineering, evolutionary analysis, and force-field calculations has been employed to enhance the catalytic activity of D-lactonohydrolase (D-Lac), which is a pivotal enzyme involved in the resolution of racemic Pantolactone during the production of vitamin B5. The best mutant, N96S/A271E/F274Y/F308G (M3), was obtained and its catalytic efficiency (k(cat)/K(M)) was nearly 23-fold higher than that of the wild-type. The M3 whole-cell converted 20 % of DL-Pantolactone into D-pantoic acid (D-PA, >99 % e.e.) with a conversion rate of 47 % and space-time yield of 107.1 g L(-1) h(-1), demonstrating its great potential for industrial-scale D-pantothenic acid production. Molecular dynamics (MD) simulations revealed that the reduction in the steric hindrance within the substrate tunnel and conformational reconstruction of the distal loop resulted in a more favourable"catalytic" conformation, making it easier for the substrate and enzyme to enter their pre-reaction state. This study illustrates the potential of the distal residue on the pivotal loop at the entrance of the D-Lac substrate tunnel as a novel modification hotspot capable of reshaping energy patterns and consequently influencing the enzymatic activity.
Pitfalls in the Optimization of Conformer Populations to Maximize the Similarity between Predicted and Experimental Chiroptical Spectra.[Pubmed:38154123]
J Phys Chem A. 2024 Jan 11;128(1):129-138.
The conformational populations of Pantolactone, epichlorohydrin, and N-acetyl-tryptophan methyl ester were investigated by using similarity analysis between their calculated and experimental chiroptical spectra. By performing the analysis on Pantolactone using two different chiroptical methods, namely, vibrational circular dichroism and Raman optical activity, it was found that the optimal sets of conformers do not match between the two methods, indicating that the conformational populations obtained by optimizing the similarity between calculated and experimental spectra are unlikely to be more accurate than energy-based Boltzmann populations. Also, it was found for Pantolactone, epichlorohydrin, and N-acetyl-tryptophan methyl ester that the similarity between calculated and experimental spectra would often not vary significantly if each of the populated conformers was discarded, one at a time. This observation indicates that more than one set of conformers can provide acceptable similarity between the predicted and experimental spectra. Therefore, the correct set of conformers cannot be accurately determined by similarity analysis.
Multi-Enzymatic Cascade for Efficient Deracemization of dl-Pantolactone into d-Pantolactone.[Pubmed:37513182]
Molecules. 2023 Jul 10;28(14):5308.
d-Pantolactone is an intermediate in the synthesis of d-pantothenic acid, which is known as vitamin B(5). The commercial synthesis of d-Pantolactone is carried out through the selective resolution of dl-Pantolactone catalyzed by lactone hydrolase. In contrast to a kinetic resolution approach, the deracemization of dl-Pantolactone is a simpler, greener, and more sustainable way to obtain d-Pantolactone with high optical purity. Herein, an efficient three-enzyme cascade was developed for the deracemization of dl-Pantolactone, using l-Pantolactone dehydrogenase from Amycolatopsis methanolica (AmeLPLDH), conjugated polyketone reductase from Zygosaccharomyces parabailii (ZpaCPR), and glucose dehydrogenase from Bacillus subtilis (BsGDH). The AmeLPLDH was used to catalyze the dehydrogenated l-Pantolactone into ketoPantolactone; the ZpaCPR was used to further catalyze the ketoPantolactone into d-Pantolactone; and glucose dehydrogenase together with glucose fulfilled the function of coenzyme regeneration. All three enzymes were co-expressed in E. coli strain BL21(DE3), which served as the whole-cell biocatalyst. Under optimized conditions, 36 h deracemization of 1.25 M dl-Pantolactone d-Pantolactone led to an e.e.(p) value of 98.6%, corresponding to productivity of 107.7 g/(l.d).
Fungicidal and plant growth-promoting traits of Lasiodiplodia pseudotheobromae, an endophyte from Andrographis paniculata.[Pubmed:37426966]
Front Plant Sci. 2023 Jun 14;14:1125630.
INTRODUTION: Fungal endophytes are widespread and dwell inside plant cells for at least part of their life without producing any symptoms of infection. Distinct host plants may have different fungal endophyte rates and community compositions. Despite this, the endophytic fungi connected with the host plant and their hostile behaviors, remain unknown. METHODS: The objective of the current research was to isolate and identify endophytic fungal species from the root of Andrographis paniculata. The effects of fungal isolate APR5 on the mycelial growth of phytopathogens and the production of plant-promoting traits were assessed. RESULTS AND DISCUSSION: Endophytic fungal isolate APR5 showed higher inhibitory efficacy in dual and double plate assay against the tested phytopathogenic fungi. The scanning electron microscope analysis demonstrated that the phytopathogenic fungal hyphae were coiled by endophytes which makes them shrink and disintegrate. Further, an ethyl acetate crude extract effectively suppressed the mycelium growth of Rhizoctonia solani by 75 +/- 0.1% in an agar well diffusion assay. The fungal isolate APR5 was identified as Lasiodiplodia pseudotheobromae using the nuclear ribosomal DNA internal transcribed spacer (ITS) region and qualitatively evaluated for their capacity to produce plant growth-promoting hormones. Gas chromatography-mass spectrometry was implemented to acquire a preliminary understanding of the secondary metabolic profile of ethyl acetate crude extract. 1-octadecene, erythritol, niacin, oleic acid, phenol, Pantolactone, phenyl ethyl alcohol, p-cresol, and tbutyl hydroquinone are the metabolites analyzed in a crude extract of APR5 isolate and are reported to have antimicrobial properties.
Increasing catalytic efficiency of SceCPR by semi-rational engineering towards the asymmetric reduction of D-pantolactone.[Pubmed:37392996]
J Biotechnol. 2023 Aug 20;373:34-41.
D-Pantolactone (D-PL) is one of the important chiral intermediates in the synthesis of D-pantothenic acid. Our previous study has revealed that ketoPantolactone (KPL) reductase in Saccharomyces cerevisiae (SceCPR) could asymmetrically reduce KPL to D-PL with a relatively weak activity. In this study, engineering of SceCPR was performed using a semi-rational design to enhance its catalytic activity. Based on the computer-aided design including phylogenetic analysis and molecular dynamics simulation, Ser158, Asn159, Gln180, Tyr208, Tyr298 and Trp299 were identified as the potential sites. Semi-saturation, single and combined-site mutagenesis was performed on all six residues, and several mutants with improved enzymatic activities were obtained. Among them, the mutant SceCPR(S158A/Y298H) exhibited the highest catalytic efficiency in which the k(cat)/K(m) value is 2466.22 s(-1).mM(-1), 18.5 times higher than that of SceCPR. The 3D structural analysis showed that the mutant SceCPR(S158A/Y298H) had an expanded and increased hydrophilicity catalytic pocket, and an enhanced pi-pi interaction which could contribute to faster conversion efficiency and higher catalytic rate. The whole cell system containing SceCPR(S158A/Y298H) and glucose dehydrogenase (GDH), under the optimized condition, could reduce 490.21 mM D-PL with e.e.>== 99%, conversion rate = 98%, and the space-time yield = 382.80 g.L(-1).d(-1), which is the highest level reported so far.
Tuning an efficient Escherichia coli whole-cell catalyst expressing l-pantolactone dehydrogenase for the biosynthesis of d-(-)-pantolactone.[Pubmed:36948403]
J Biotechnol. 2023 Apr 10;367:1-10.
d-(-)-Pantolactone (DPL) is a key intermediate for the production of d-(+)-pantothenate (vitamin B5). Deracemization of d,l-Pantolactone (D,L-PL) through oxidizing l-(+)-Pantolactone (LPL) to ketopantoyl lactone (KPL) and subsequently reducing KPL to DPL is a promising route for synthesizing DPL. Herein, a newly mined l-Pantolactone dehydrogenase from Rhodococcus hoagie (RhoLPLDH) was used for the oxidative dehydrogenation of LPL. To alleviate inclusion bodies formed by membrane-bound RhoLPLDH intracellular expression in E. coli, strategies involving chaperone assistance and decreasing induction temperature were used to achieve RhoLPLDH soluble expression. To enhance its activity, directed evolution and hydrophilicity-based engineering yielded increased catalytic activity and thermostability. 1 M LPL was efficiently converted to KPL by engineering strain CM5 co-expressing RhoLPLDH(L254I/V241I/I156L/F224Q/N164K) and chaperone. A "two stages in one-pot" method was employed in deracemization of 1 M D,L-PL with 91.2% yield. These results demonstrated that CM5 catalyst exhibits great potential in enzyme cascade deracemization for the production of DPL.
Industrial kinetic resolution of d,l-pantolactone by an immobilized whole-cell biocatalyst.[Pubmed:35480294]
RSC Adv. 2021 Sep 13;11(48):30373-30376.
Immobilized whole-cells of Pichia pastoris harboring recombinant d-lactonase were entrapped in calcium alginate gels and used as an efficient biocatalyst for catalytic kinetic resolution of d,l-Pantolactone. The immobilized whole-cell biocatalyst exhibited good catalytic stability, which was applied for stereospecific hydrolysis of d-Pantolactone for up to 56 repeated batch reactions without obvious loss in the catalytic activity and enantioselectivity.
Engineering precursor and co-factor supply to enhance D-pantothenic acid production in Bacillus megaterium.[Pubmed:35175424]
Bioprocess Biosyst Eng. 2022 May;45(5):843-854.
High-yielding chemical and chemo-enzymatic methods of D-pantothenic acid (DPA) synthesis are limited by using poisonous chemicals and DL-Pantolactone racemic mixture formation. Alternatively, the safe microbial fermentative route of DPA production was found promising but suffered from low productivity and precursor supplementation. In this study, Bacillus megaterium was metabolically engineered to produce DPA without precursor supplementation. In order to provide a higher supply of precursor D-pantoic acid, key genes involved in its synthesis are overexpressed, resulting strain was produced 0.53 +/- 0.08 g/L DPA was attained in shake flasks. Cofactor CH2-THF was found to be vital for DPA biosynthesis and was regenerated through the serine-glycine degradation pathway. Enhanced supply of another precursor, beta-alanine was achieved by codon optimization and dosing of the limiting L-asparate-1-decarboxylase (ADC). Co-expression of Pantoate-beta-alanine ligase, ADC, phosphoenolpyruvate carboxylase, aspartate aminotransferase and aspartate ammonia-lyase enhanced DPA concentration to 2.56 +/- 0.05 g/L at shake flasks level. Fed-batch fermentation in a bioreactor with and without the supplementation of beta-alanine increased DPA concentration to 19.52 +/- 0.26 and 4.78 +/- 0.53 g/L, respectively. This present study successfully demonstrated a rational approach combining precursor supply engineering with cofactor regeneration for the enhancement of DPA titer in recombinant B. megaterium.
Biocatalysis of heterogenously-expressed d-lactonohydrolases and its efficient preparation of desirable d-pantoic acid.[Pubmed:35007923]
Enzyme Microb Technol. 2022 Apr;155:109981.
d-Pantoic acid (D-PA) is an essential intermediate for the production of d-Pantolactone. Here, three d-lactonohydrolases (D-Lacs), namely, Fm-Lac from Fusarium moniliforme SW-902, Fp-Lac from Fusarium proliferatum Nirenberg ECU2002, and Fo-Lac from Fusarium oxysporum AKU3702 were heterogeneously expressed in Pichia pastoris. The constructed recombinant strains produced D-Lacs of 1263 U/mL, 1025 U/mL, and 948 U/mL in a 3-L fermenter, respectively. Simultaneously, these three D-Lacs were used to resolve racemic Pantolactone (DL-PL), the hydrolysis rate by Fo-Lac over 40% and the enantiomeric excesses was 99% after 4 h reaction, which outperformed Fm-Lac and Fp-Lac. Under the 800 mL scale reaction, the hydrolysis rate of DL-PL reached 39.2% with a D-PA concentration of 144.6 g/L and space-time yield of 36.2 g/L/h correspondingly. This is the highest catalytic efficiency reported so far, which shows that D-Lac heterologously expressed by P. pastoris has excellent industrial application prospects.
Chiral analysis of pantolactone with molecular rotational resonance spectroscopy.[Pubmed:34698412]
Chirality. 2022 Jan;34(1):114-125.
A molecular rotational resonance spectroscopy method for measuring the enantiomeric excess of Pantolactone, an intermediate in the synthesis of panthenol and pantothenic acid, is presented. The enantiomers are distinguished via complexation with a small chiral tag molecule, which produces diastereomeric complexes in the pulsed jet expansion used to inject the sample into the spectrometer. These complexes have distinct moments of inertia, so their spectra are resolved by MRR spectroscopy. Quantitative enantiomeric excess (EE) measurements are made by taking the ratio of normalized complex signal levels when a chiral tag sample of high, known EE is used, while the absolute configuration of the sample can be determined from electronic structure calculations of the complex geometries. These measurements can be performed without the need for reference samples with known enantiopurity. Two instruments were used in the analysis. A broadband, chirped-pulse spectrometer is used to perform structural characterization of the complexes. The broadband spectrometer is also used to determine the EE; however, this approach requires relatively long measurement times. A targeted MRR spectrometer is also used to demonstrate EE analysis with approximately 15-min sample-to-sample cycle time. The quantitative accuracy of the method is demonstrated by comparison with chiral gas chromatography and through the measurement of a series of reference samples prepared from mixtures of (R)-Pantolactone and (S)-Pantolactone samples of known EE.
Asymmetric synthesis of pharmaceutically relevant 1-aryl-2-heteroaryl- and 1,2-diheteroarylcyclopropane-1-carboxylates.[Pubmed:34522315]
Chem Sci. 2021 Jul 27;12(33):11181-11190.
This study describes general methods for the enantioselective syntheses of pharmaceutically relevant 1-aryl-2-heteroaryl- and 1,2-diheteroarylcyclopropane-1-carboxylates through dirhodium tetracarboxylate-catalysed asymmetric cyclopropanation of vinyl heterocycles with aryl- or heteroaryldiazoacetates. The reactions are highly diastereoselective and high asymmetric induction could be achieved using either (R)-Pantolactone as a chiral auxiliary or chiral dirhodium tetracarboxylate catalysts. For meta- or para-substituted aryl- or heteroaryldiazoacetates the optimum catalyst was Rh(2)(R-p-Ph-TPCP)(4). In the case of ortho-substituted aryl- or heteroaryldiazoacetates, the optimum catalyst was Rh(2)(R-TPPTTL)(4). For a highly enantioselective reaction with the ortho-substituted substrates, 2-chloropyridine was required as an additive in the presence of either 4 A molecular sieves or 1,1,1,3,3,3-hexafluoroisopropanol (HFIP). Under the optimized conditions, the cyclopropanation could be conducted in the presence of a variety of heterocycles, such as pyridines, pyrazines, quinolines, indoles, oxadiazoles, thiophenes and pyrazoles.
Enantioenriched Positive Allosteric Modulators Display Distinct Pharmacology at the Dopamine D(1) Receptor.[Pubmed:34206465]
Molecules. 2021 Jun 22;26(13):3799.
(1) Background: Two first-in-class racemic dopamine D(1) receptor (D(1)R) positive allosteric modulator (PAM) chemotypes (1 and 2) were identified from a high-throughput screen. In particular, due to its selectivity for the D(1)R and reported lack of intrinsic activity, compound 2 shows promise as a starting point toward the development of small molecule allosteric modulators to ameliorate the cognitive deficits associated with some neuropsychiatric disease states; (2) Methods: Herein, we describe the enantioenrichment of optical isomers of 2 using chiral auxiliaries derived from (R)- and (S)-3-hydroxy-4,4-dimethyldihydrofuran-2(3H)-one (d- and l-Pantolactone, respectively); (3) Results: We confirm both the racemate and enantiomers of 2 are active and selective for the D(1)R, but that the respective stereoisomers show a significant difference in their affinity and magnitude of positive allosteric cooperativity with dopamine; (4) Conclusions: These data warrant further investigation of asymmetric syntheses of optically pure analogues of 2 for the development of D(1)R PAMs with superior allosteric properties.
Effect of 2-propanol content on solute retention mechanisms determined using amylose tris(3,5-dimethylphenylcarbamate) chiral stationary phase under normal- and reversed-phase conditions.[Pubmed:34087518]
J Chromatogr A. 2021 Aug 2;1650:462226.
The electrostatic interactions between chiral solutes and polysaccharide (PS)-based chiral selectors are the key to achieving chiral recognition; however, PS-based sorbents, derivatized of phenyl moieties, can exhibit considerably non-polar characteristics, and they are also useful for the separation of enantiomers in the reversed-phase mode. In this study, an immobilized amylose 3,5-dimethylphenylcarbamate-based sorbent was used to investigate the balance between electrostatic interactions and solvophobic interactions, with complementary effects on solute retention behavior when the isopropanol (IPA) concentration was altered. It was proposed that in both normal- and reversed-phase modes, information on the retention mechanisms could be obtained by observing the curvature of the logarithm of the retention factor versus the logarithm of the IPA concentration, and the slope values of the curves were related to the number of displaced IPA molecules upon solute adsorption. Using the proposed model and the two-site adsorption model, the retention behaviors of Pantolactone (PL) enantiomers in both normal- and reversed-phase modes were investigated. The PL-sorbent interactions were classified into four types: electrostatic/enantioselective, electrostatic/nonselective, solvophobic/enantioselective, and solvophobic/nonselective. At IPA concentrations below 50 vol.% in n-hexane, the retention behaviors of PL were dominated by electrostatic/enantioselective sites, whereas at IPA concentrations beyond 50 vol.%, the solvophobic interactions of PL-sorbent were strengthened and mostly nonselective. By contrast, in the reversed-phase mode, a reverse in the enantiomeric elution order of PL was observed at 10 vol.% IPA, and considerably different enantioselectivity behaviors were found below and above 20 vol.%, indicating an abrupt change in the sorbent molecular environment. At IPA concentrations beyond 40 vol.%, the presence of PL-sorbent electrostatic interactions enhanced chiral recognition.