Dihydroxyfumaric acidCAS# 133-38-0 |
Quality Control & MSDS
Number of papers citing our products
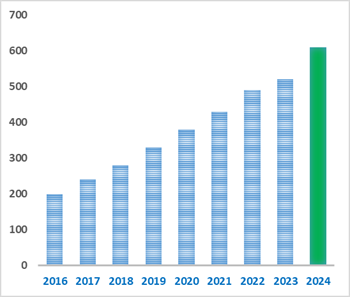
Chemical structure

Cas No. | 133-38-0 | SDF | Download SDF |
PubChem ID | N/A | Appearance | Powder |
Formula | C4H4O6 | M.Wt | 148.0 |
Type of Compound | Miscellaneous | Storage | Desiccate at -20°C |
Solubility | Soluble in Chloroform,Dichloromethane,Ethyl Acetate,DMSO,Acetone,etc. | ||
General tips | For obtaining a higher solubility , please warm the tube at 37 ℃ and shake it in the ultrasonic bath for a while.Stock solution can be stored below -20℃ for several months. We recommend that you prepare and use the solution on the same day. However, if the test schedule requires, the stock solutions can be prepared in advance, and the stock solution must be sealed and stored below -20℃. In general, the stock solution can be kept for several months. Before use, we recommend that you leave the vial at room temperature for at least an hour before opening it. |
||
About Packaging | 1. The packaging of the product may be reversed during transportation, cause the high purity compounds to adhere to the neck or cap of the vial.Take the vail out of its packaging and shake gently until the compounds fall to the bottom of the vial. 2. For liquid products, please centrifuge at 500xg to gather the liquid to the bottom of the vial. 3. Try to avoid loss or contamination during the experiment. |
||
Shipping Condition | Packaging according to customer requirements(5mg, 10mg, 20mg and more). Ship via FedEx, DHL, UPS, EMS or other couriers with RT, or blue ice upon request. |
Description | Dihydroxyfumaric acid can induce lipid peroxidation in rat liver microsomes. |

Dihydroxyfumaric acid Dilution Calculator

Dihydroxyfumaric acid Molarity Calculator
1 mg | 5 mg | 10 mg | 20 mg | 25 mg | |
1 mM | 6.7568 mL | 33.7838 mL | 67.5676 mL | 135.1351 mL | 168.9189 mL |
5 mM | 1.3514 mL | 6.7568 mL | 13.5135 mL | 27.027 mL | 33.7838 mL |
10 mM | 0.6757 mL | 3.3784 mL | 6.7568 mL | 13.5135 mL | 16.8919 mL |
50 mM | 0.1351 mL | 0.6757 mL | 1.3514 mL | 2.7027 mL | 3.3784 mL |
100 mM | 0.0676 mL | 0.3378 mL | 0.6757 mL | 1.3514 mL | 1.6892 mL |
* Note: If you are in the process of experiment, it's necessary to make the dilution ratios of the samples. The dilution data above is only for reference. Normally, it's can get a better solubility within lower of Concentrations. |
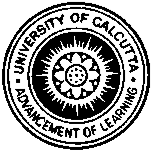
Calcutta University
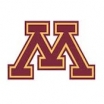
University of Minnesota
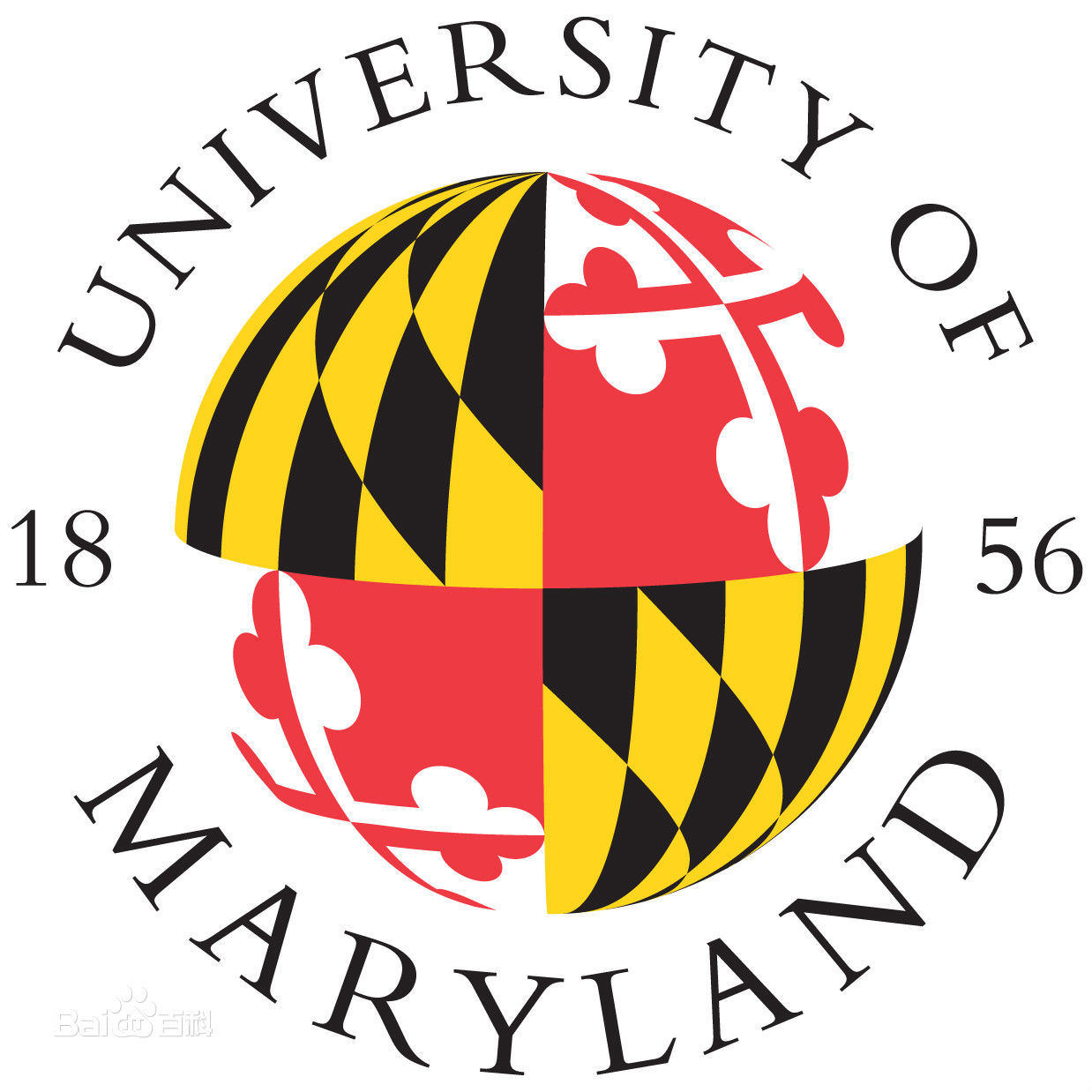
University of Maryland School of Medicine
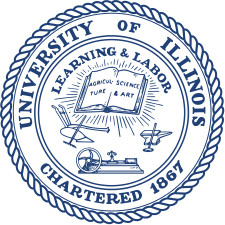
University of Illinois at Chicago
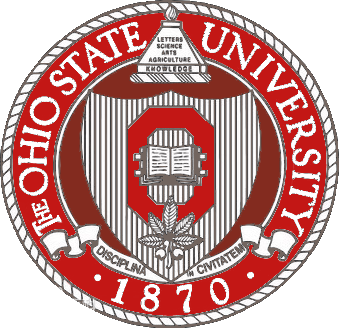
The Ohio State University
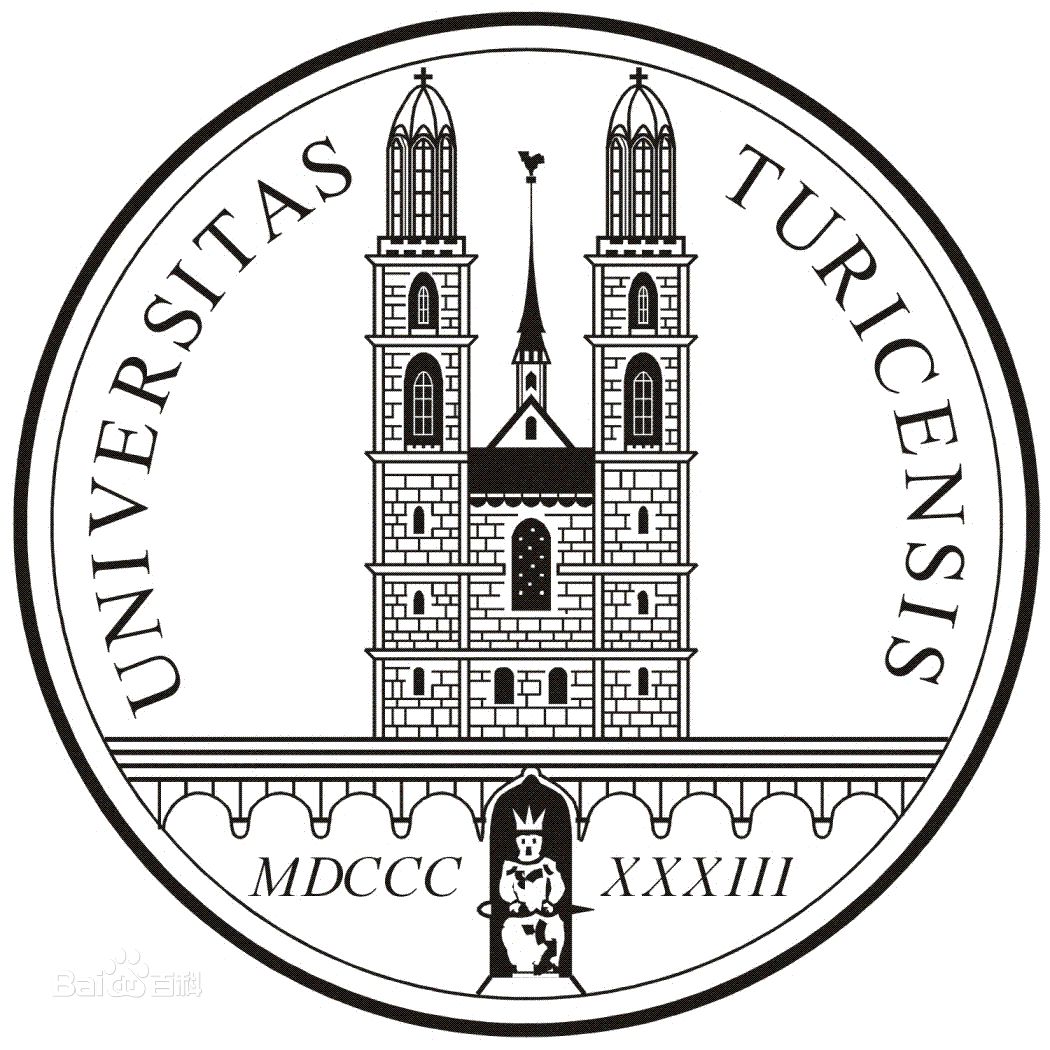
University of Zurich
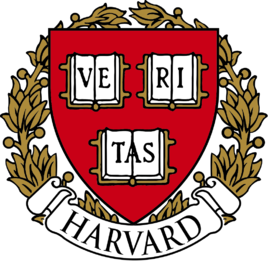
Harvard University
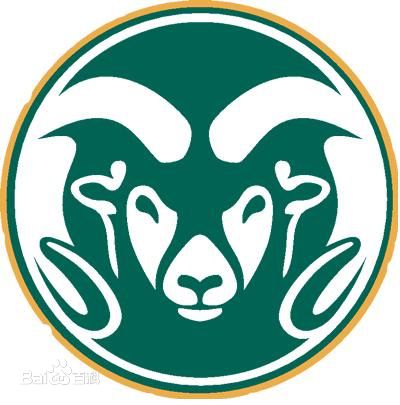
Colorado State University
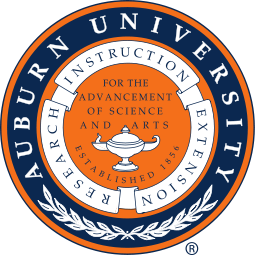
Auburn University
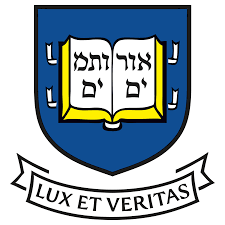
Yale University
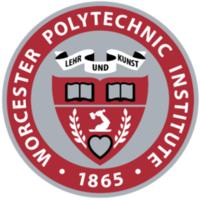
Worcester Polytechnic Institute
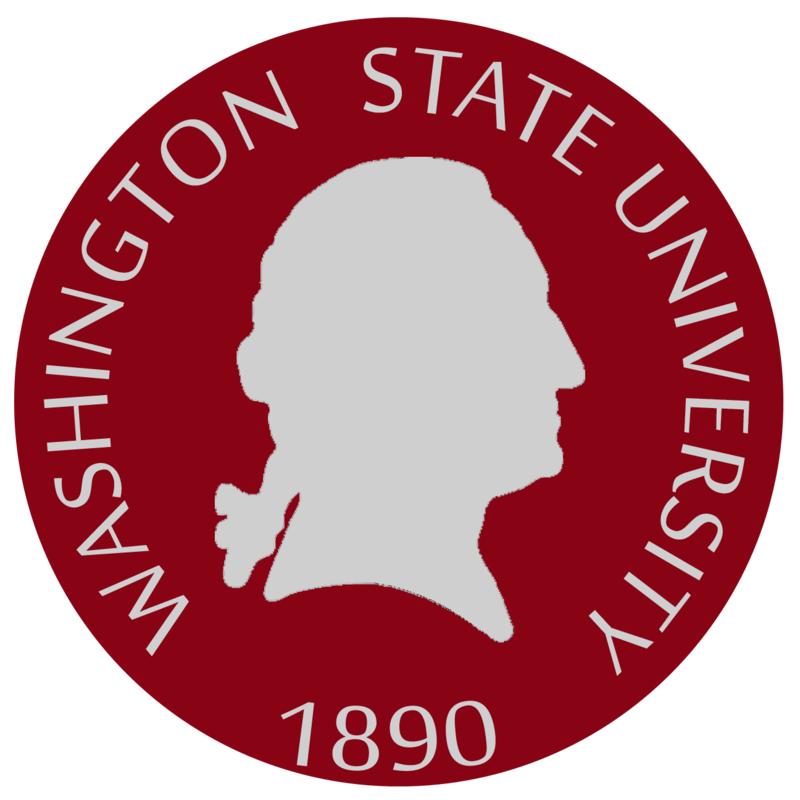
Washington State University
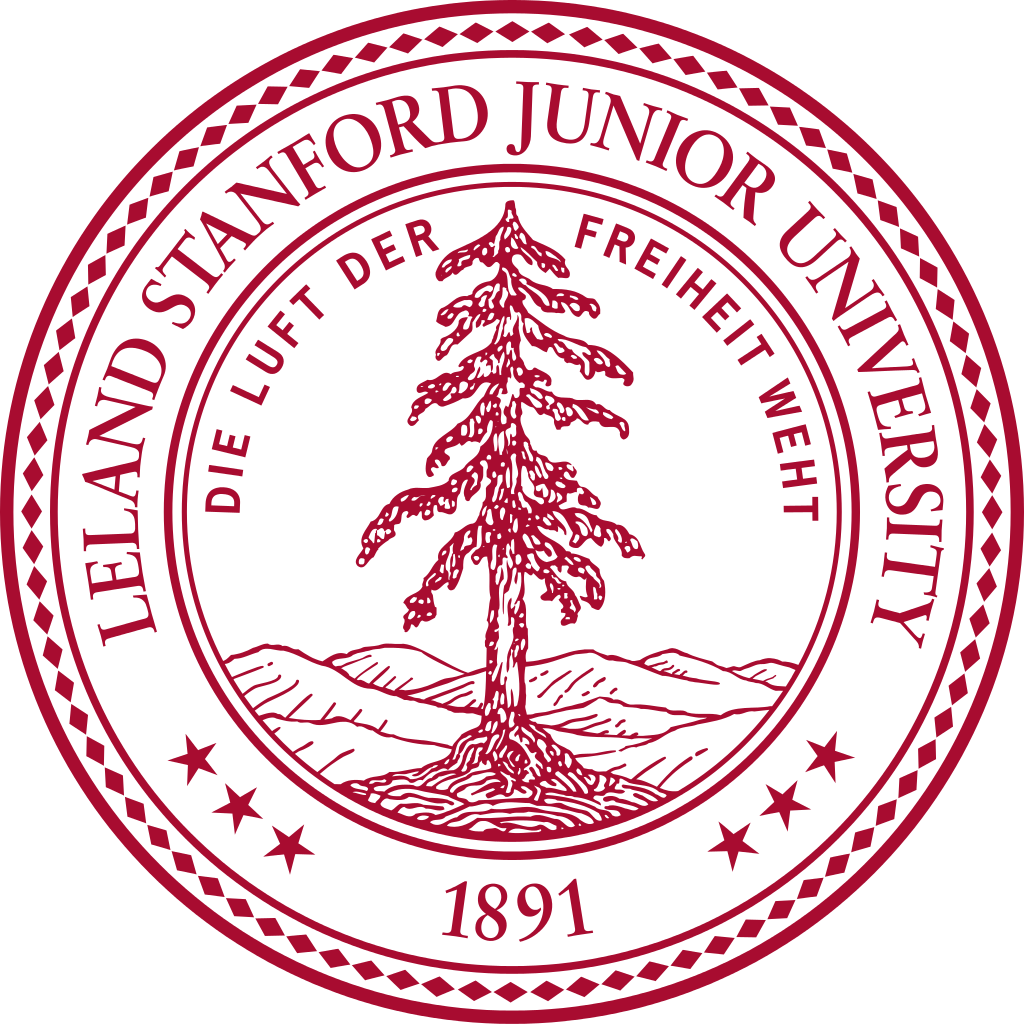
Stanford University
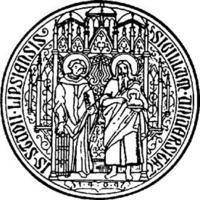
University of Leipzig
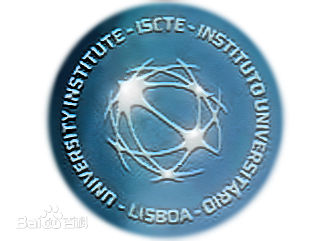
Universidade da Beira Interior
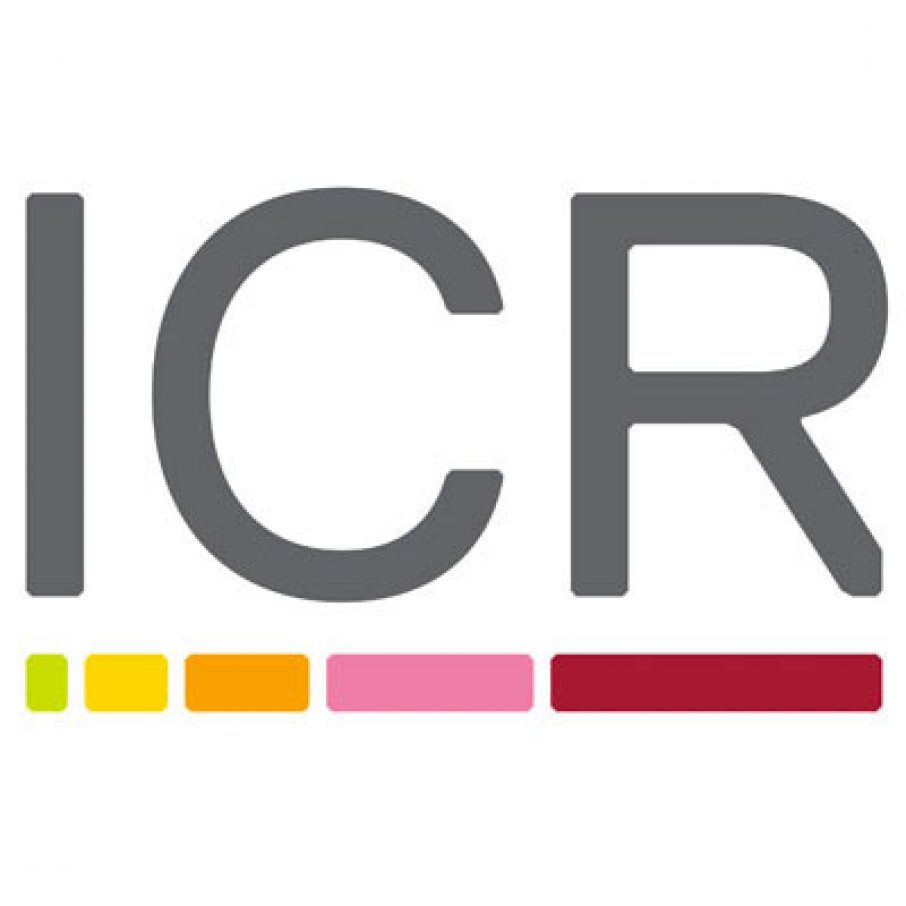
The Institute of Cancer Research
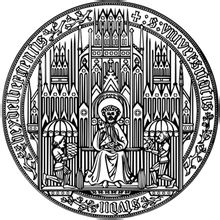
Heidelberg University
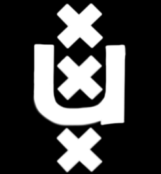
University of Amsterdam
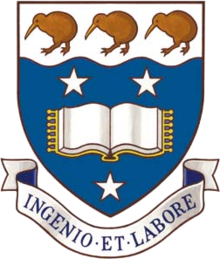
University of Auckland
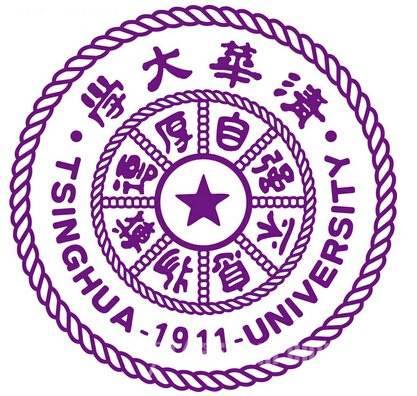
TsingHua University
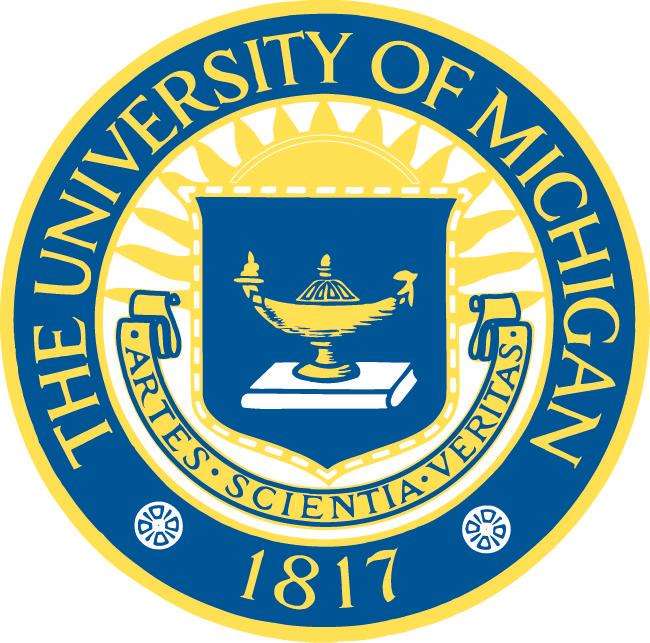
The University of Michigan
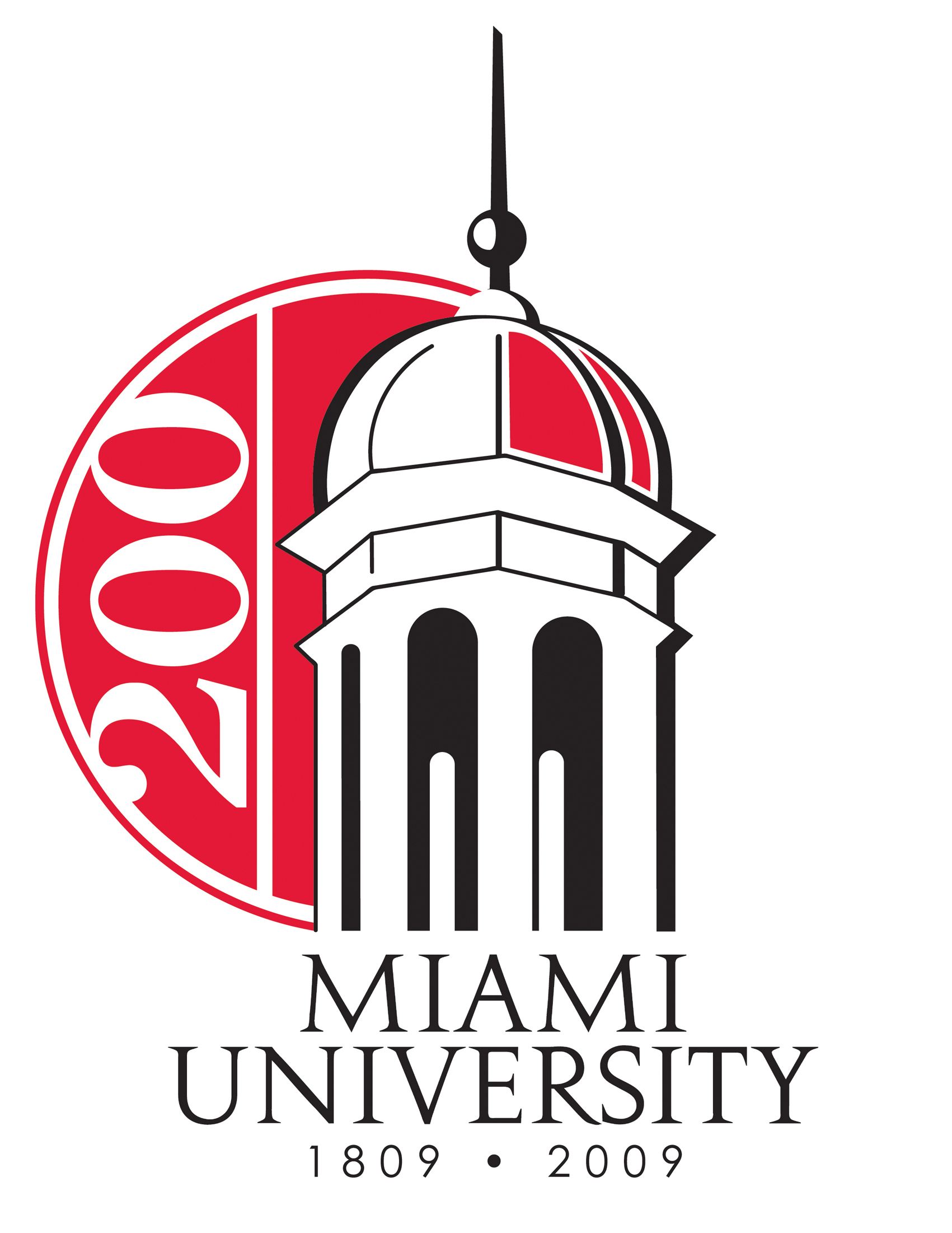
Miami University
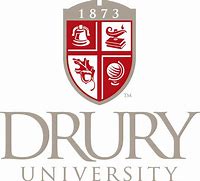
DRURY University
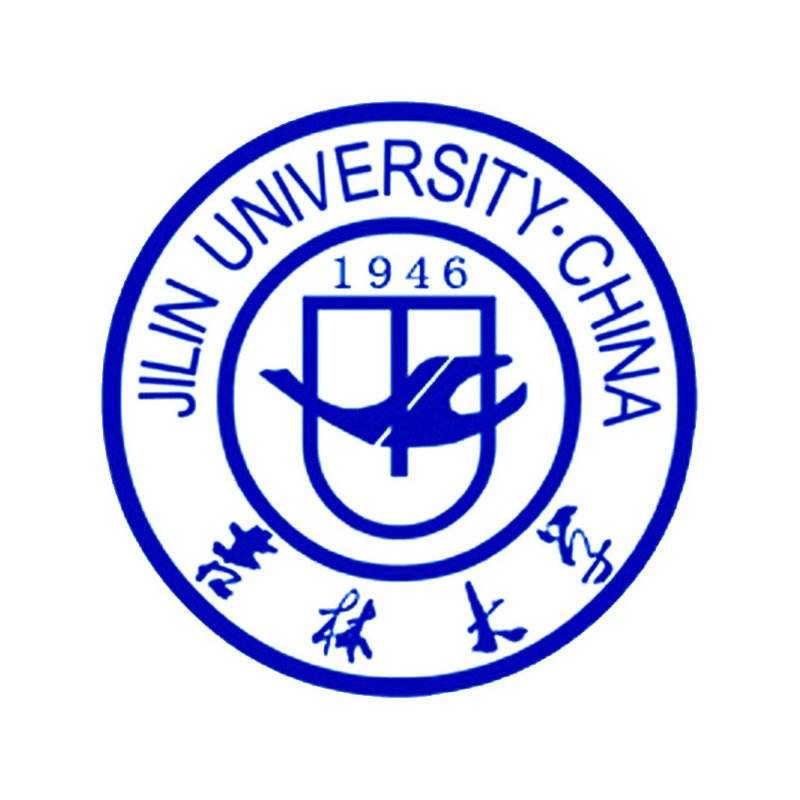
Jilin University
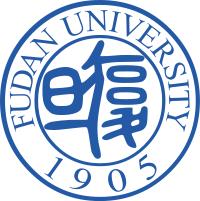
Fudan University
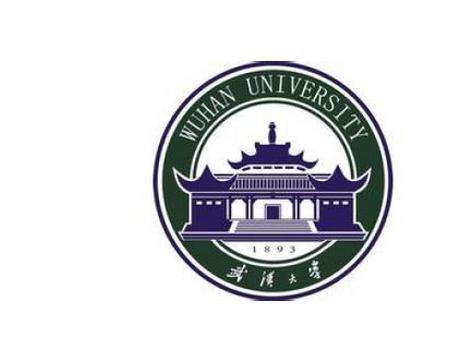
Wuhan University
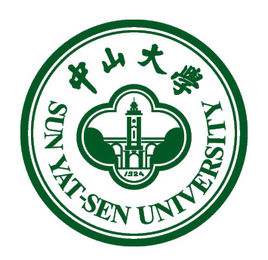
Sun Yat-sen University
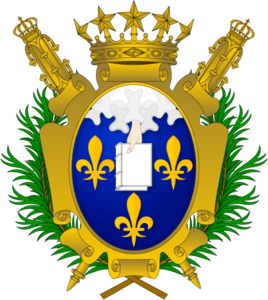
Universite de Paris
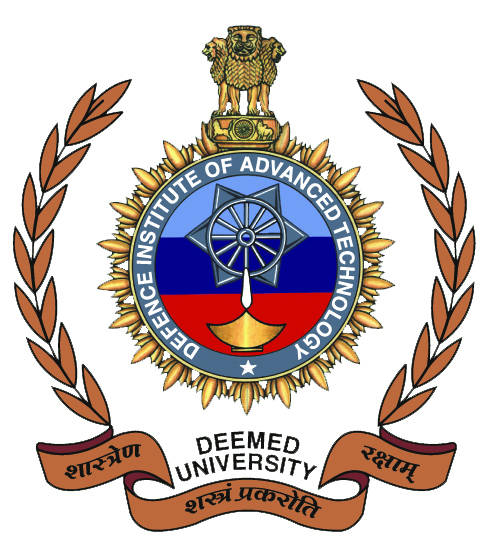
Deemed University
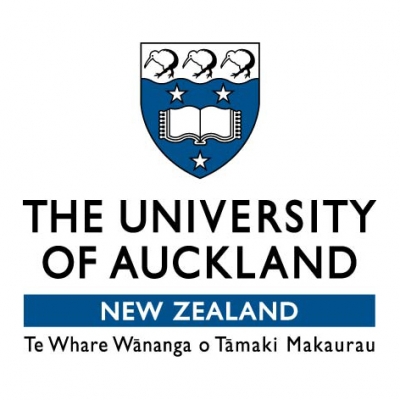
Auckland University
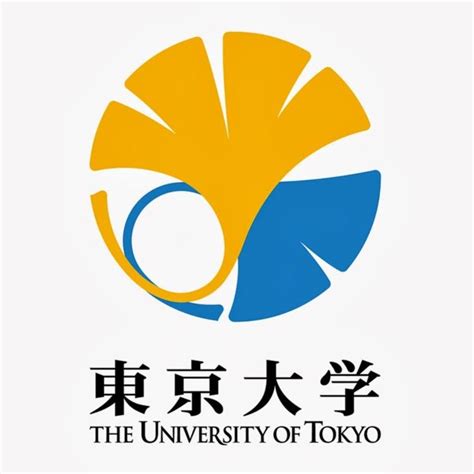
The University of Tokyo
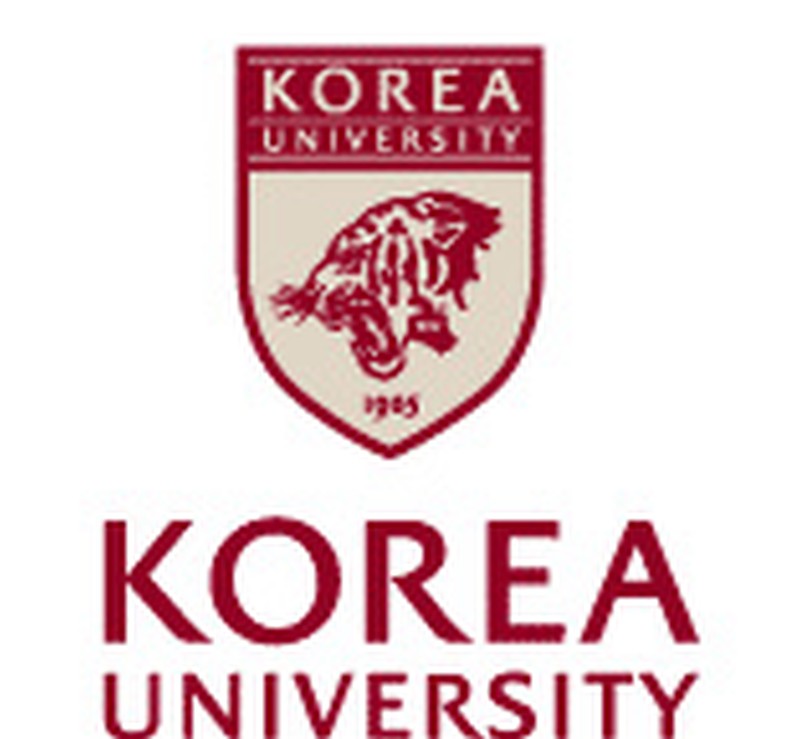
Korea University
- Apigenin 4'-O-(2'',6''-di-O-E-p-coumaroyl)glucoside
Catalog No.:BCN0059
CAS No.:71781-79-8
- Myosmine
Catalog No.:BCN0058
CAS No.:532-12-7
- Hippuric acid
Catalog No.:BCN0057
CAS No.:495-69-2
- 3-Ethoxy-4-hydroxybenzaldehyde
Catalog No.:BCN0056
CAS No.:121-32-4
- 2-Nonyl alcohol
Catalog No.:BCN0055
CAS No.:628-99-9
- 6-Hydroxyflavanone
Catalog No.:BCN0054
CAS No.:4250-77-5
- (S)-(-)-3,7-Dimethyl-6-octenal
Catalog No.:BCN0053
CAS No.:5949-05-3
- Miliacin
Catalog No.:BCN0052
CAS No.:5945-45-9
- N1,N5,N10-(E)-tri-p-coumaroylspermidine
Catalog No.:BCN0051
CAS No.:364368-18-3
- Gossypetin 3,3',8-trimethylether
Catalog No.:BCN0050
CAS No.:14965-08-3
- (S)-(-)-beta-Citronellol
Catalog No.:BCN0049
CAS No.:7540-51-4
- Chrysantellin A
Catalog No.:BCN0048
CAS No.:73039-13-1
- 4-Isopropylbenzaldehyde
Catalog No.:BCN0061
CAS No.:122-03-2
- 2',6'-Dihydroxy-4,4'-dimethoxychalcone
Catalog No.:BCN0062
CAS No.:20621-49-2
- Acetic acid m-cresyl ester
Catalog No.:BCN0063
CAS No.:122-46-3
- Kaempferol 3,4'-diglucoside 7-rhamnoside
Catalog No.:BCN0064
CAS No.:1131009-93-2
- Delta-Nonalactone
Catalog No.:BCN0065
CAS No.:3301-94-8
- 1,4-Cineole
Catalog No.:BCN0066
CAS No.:470-67-7
- (R)-(+)-Limonene
Catalog No.:BCN0067
CAS No.:5989-27-5
- Undecanolactone
Catalog No.:BCN0068
CAS No.:710-04-3
- Laricitrin
Catalog No.:BCN0069
CAS No.:53472-37-0
- Peucenidin
Catalog No.:BCN0070
CAS No.:33044-93-8
- Homobutein
Catalog No.:BCN0071
CAS No.:34000-39-0
- Undecanoic gamma-lactone
Catalog No.:BCN0072
CAS No.:104-67-6
Base-Mediated Cascade Aldol Addition and Fragmentation Reactions of Dihydroxyfumaric Acid and Aromatic Aldehydes: Controlling Chemodivergence via Choice of Base, Solvent, and Substituents.[Pubmed:30223647]
J Org Chem. 2018 Dec 7;83(23):14219-14233.
The diester derivative of Dihydroxyfumaric acid (DHF) has been used exclusively as an electrophile in organic synthesis. However, the synthetic utility of DHF's nucleophilic reactivity, contained in the ene-diol moiety, has been underexplored. Inspired by recently observed pH-dependent chemodivergent nucleophilic aldol reactions of dihydroxyfumarate (DHF(2-)) with glyoxylate and formaldehyde, we report herein the control and synthetic application of base-controlled chemodivergent reactions between dihydroxyfumarate and aromatic and heteroaromatic aldehydes. With hydroxide as the base in a predominantly aqueous medium, aldol addition followed by deoxalation occurs to provide various 3-aryl-2,3-dihydroxypropanoic acids. With triethylamine as the base in THF, 1-aryl-2,3-dihydroxypropanones are the products of the reaction. In order to understand the difference in reactivity between DHF, its dicarboxylate, and its dimethyl ester, we undertook computational and experimental studies that provide a rationale as to why the dihydroxyfumarate (DHF(2-)) is a nucleophile while the corresponding diester reacts as an electrophile.
Decarboxylative Cascade Reactions of Dihydroxyfumaric Acid: A Preparative Approach to the Glyoxylate Scenario.[Pubmed:27270049]
Org Lett. 2016 Jun 17;18(12):2950-3.
An operationally simple protocol is reported to generate an alpha-hydroxyacyl anion by the decarboxylation of Dihydroxyfumaric acid. To date, the "missing" utilization of the hydroxyacyl anion in highly chemo- and stereoselective cascade reactions enables short and direct construction of carbohydrates.
A heme peroxidase with a functional role as an L-tyrosine hydroxylase in the biosynthesis of anthramycin.[Pubmed:21919439]
Biochemistry. 2011 Oct 18;50(41):8926-36.
We report the first characterization and classification of Orf13 (S. refuineus) as a heme-dependent peroxidase catalyzing the ortho-hydroxylation of L-tyrosine to L-DOPA. The putative tyrosine hydroxylase coded by orf13 of the anthramycin biosynthesis gene cluster has been expressed and purified. Heme b has been identified as the required cofactor for catalysis, and maximal L-tyrosine conversion to L-DOPA is observed in the presence of hydrogen peroxide. Preincubation of L-tyrosine with Orf13 prior to the addition of hydrogen peroxide is required for L-DOPA production. However, the enzyme becomes inactivated by hydrogen peroxide during catalysis. Steady-state kinetic analysis of L-tyrosine hydroxylation revealed similar catalytic efficiency for both L-tyrosine and hydrogen peroxide. Spectroscopic data from a reduced-CO(g) UV-vis spectrum of Orf13 and electron paramagnetic resonance of ferric heme Orf13 are consistent with heme peroxidases that have a histidyl-ligated heme iron. Contrary to the classical heme peroxidase oxidation reaction with hydrogen peroxide that produces coupled aromatic products such as o,o'-dityrosine, Orf13 is novel in its ability to catalyze aromatic amino acid hydroxylation with hydrogen peroxide, in the substrate addition order and for its substrate specificity for L-tyrosine. Peroxygenase activity of Orf13 for the ortho-hydroxylation of L-tyrosine to L-DOPA by a molecular oxygen dependent pathway in the presence of Dihydroxyfumaric acid is also observed. This reaction behavior is consistent with peroxygenase activity reported with horseradish peroxidase for the hydroxylation of phenol. Overall, the putative function of Orf13 as a tyrosine hydroxylase has been confirmed and establishes the first bacterial class of tyrosine hydroxylases.
VO2+ complexation by bioligands showing keto-enol tautomerism: a potentiometric, spectroscopic, and computational study.[Pubmed:21902189]
Inorg Chem. 2011 Oct 17;50(20):10328-41.
The interaction of VO(2+) ion with ligands of biological interest that are present in important metabolic pathways--2-oxopropanoic acid (pyruvic acid, pyrH), 3-hydroxy-2-oxopropanoic acid (3-hydroxypyruvic acid, hydpyrH), oxobutanedioic acid (oxalacetic acid, oxalH(2)), (S)-hydroxybutanedioic acid (l-malic acid, malH(2)), and 2,3-dihydroxy-(E)-butanedioic acid (Dihydroxyfumaric acid, dhfH(2))--was described. Their complexing capability was compared with that of similar ligands: 3-hydroxy-2-butanone (hydbut) and 3,4-dihydroxy-3-cyclobutene-1,2-dione (squaric acid, squarH(2)). All of these ligands (except l-malic acid) exhibit keto-enol tautomerism, and the presence of a metal ion can influence such an equilibrium. The different systems were studied with electron paramagnetic resonance (EPR) and UV-vis spectroscopies and with pH potentiometry. Density functional theory (DFT) methods provide valuable information on the relative energy of the enol and keto forms of the ligands both in the gas phase and in aqueous solution, on the geometry of the complexes, and on EPR and electronic absorption parameters. The results show that most of the ligands behave like alpha-hydroxycarboxylates, forming mono- and bis-chelated species with (COO(-), O(-)) coordination, demonstrating that the metal ion is able to stabilize the enolate form of some ligands. With Dihydroxyfumaric acid, the formation of a non-oxidovanadium(IV) complex, because of rearrangement of dihydroxyfumaric to dihydroxymaleic acid (dhmH(2)), can be observed. With 3-hydroxy-2-butanone and 3,4-dihydroxy-3-cyclobutene-1,2-dione, complexation of VO(2+) does not take place and the reason for this behavior is explained by chemical considerations and computational calculations.
Diastereoselective self-condensation of dihydroxyfumaric acid in water: potential route to sugars.[Pubmed:21748829]
Angew Chem Int Ed Engl. 2011 Aug 22;50(35):8127-30.
Jack of all trades: water-soluble salts of DHF underwent self-condensation to afford the threo diastereomer of pentulosonic acid, through differing reaction pathways contingent on the metal salt used. This transformation exemplifies the diverging roles of DHF as a nucleophile (a synthon for alpha-hydroxyacetyl anion) and an electrophile (an alpha-carboxyglycolaldehyde equivalent).
Facile synthesis of anion dependent versatile Cu(I) and mixed-valent porous Cu(I)/Cu(II) frameworks.[Pubmed:20333332]
Dalton Trans. 2010 Apr 14;39(14):3412-9.
CuX(2) (X = ClO(4)(-), NO(3)(-), 0.5 SO(4)(2-), Cl(-)) and 2,3-Dihydroxyfumaric acid (dhfa) undergo redox reaction in slightly basic aqueous solution forming Cu(I)X and 2,3-diketosuccinic acid. Pyrazine (pyz) and 1,2-bis(4-pyridyl)ethylene (bpee) linkers have been used for stabilizing and isolating the Cu(I) state in this reaction. The three different Cu(I)-frameworks, {Cu(pyz)(1.5)(ClO(4))}(n) (1), {Cu(pyz)(NO(3))}(n) (2), and {[Cu(bpee)Cl].2H(2)O}(n) (3) with different anions have been synthesized and structurally characterized. The framework topology and dimensionality depends upon the anions. This is a one step synthesis of Cu(I) coordination frameworks with 1 : 2 stoichiometry in relation to dhfa and Cu(II). By controlling the concentration of dhfa, a mixed-valent porous Cu(I)/Cu(II) framework, [Cu(II)Cu(I)(2)(2,4-pyrdc)(2)(pyz)(3)].2H(2)O}(n) (4) has been synthesized and characterized by XPS and magnetic studies. Dehydrated frameworks of 3 and 4 exhibit interesting sorption properties with different solvent molecules. In a similar reaction CuCl(2) and pyz without dhfa produced the corresponding Cu(II)-compound, {Cu(pyz)Cl(2)}(n) (5), suggesting the reducing role of dhfa. Therefore by this simple reaction, it is possible to control the oxidation state as well as the coordination number and geometry of Cu ions. This is a novel synthetic methodology for the stabilization of the Cu +1 state in aqueous solution and open atmosphere.
Two- and three-dimensional hydrated coordination polymers of diaqualanthanum(3+) ions with 2-hydroxypropanedioate, oxalate and acetate anions as bridging ligands.[Pubmed:19346602]
Acta Crystallogr C. 2009 Apr;65(Pt 4):m160-4.
The title compounds, poly[[tetraaquadi-mu-2-hydroxypropanedioato-mu-oxalato-dilanthanum(III)] tetrahydrate], {[La(2)(C(2)O(4))(C(3)H(2)O(5))(2)(H(2)O)(4)].4H(2)O}(n), (I), and poly[[tetra-mu-acetato-tetraaqua-mu-oxalato-dilanthanum(III)] dihydrate], {[La(2)(C(2)O(4))(C(2)H(3)O(2))(4)(H(2)O)(4)].2H(2)O}(n), (II), represent crystalline hydrates of coordination polymers of diaqualanthanum(3+) ions with different combinations of bridging carboxylate ligands, viz. 2-hydroxypropanedioate and oxalate in a 2:2:1 ratio in (I), and acetate and oxalate in a 2:4:1 ratio in (II). While the acetate component was one of the reactants used, the other ligands were obtained in situ by aerial oxidation of the Dihydroxyfumaric acid present in the reactions. The crystal structure of (I) consists of two-dimensional polymeric arrays with water molecules intercalated between and hydrogen bonded to the arrays. The oxalate components are located on inversion centres. The crystal structure of (II) reveals an open three-dimensional polymeric connectivity between the interacting components, with the solvent water molecules incorporated within the intralattice voids and hydrogen bonded to the polymeric framework. The La(III) ion and the noncoordinated water molecules are located on axes of twofold symmetry. The oxalate group is centred at the 222 symmetry site, the intersection of the three rotation axes. The coordination number of the La(III) ion in the two structures is 10. The significance of this study lies mainly in the characterization of two new coordination polymers, as well as in the confirmation that Dihydroxyfumaric acid tends to rearrange to form smaller components in standard laboratory procedures, and should be considered a poor reagent for formulating hybrid coordination polymers with metal ions.
Kinetic study of the hexacyanoferrate (III) oxidation of dihydroxyfumaric acid in acid media.[Pubmed:18473446]
J Phys Chem A. 2008 Jun 5;112(22):4921-8.
The kinetics of the hexacyanoferrate (III) oxidation of Dihydroxyfumaric acid to hexacyanoferrate (II) and diketosuccinic acid was looked into within the 0.04 to 5.3 M HCl acidity range under different temperatures, ionic strengths, and solvent permittivity conditions. The kinetic effect of alkali metal ions, transition metal impurities, and substrate concentrations have also been analyzed. The observed inhibition effect brought about by addition of the reaction product, hexacyanoferrate (II), is a sign of a complex mechanism. The rate constants remained essentially unchanged up to 1 M HCl, diminished between 1.0 and 3.0 M HCl, and rose above 3.0 M HCl. Depending on the medium acidity, three mechanisms can be put forward, which involve different kinetically active forms. At low acidity, the rate-determining step involves a radical cation and both the neutral and the anion substrate forms are equally reactive ( k 1 = k 2 = 2.18 +/- 0.05 M (-1) s (-1), k -1 = 0.2 +/- 0.03). When the medium acidity is boosted, the rate-determining step involves the neutral Dihydroxyfumaric acid and two hexacyanoferrate (III) forms. In the intermediate region the rate constant diminished with rising [H (+)] ( k' 1 = 0.141 +/- 0.01 and k' 2 = 6.80 +/- 0.05). Specific catalytic effect by binding of alkali metal ions to oxidant has not been observed. In all instances it was assessed that the substrate decomposition is slow compared to the redox reaction.
Endogenous formation of N'-nitrosonornicotine in F344 rats in the presence of some antioxidants and grape seed extract.[Pubmed:17637060]
J Agric Food Chem. 2007 Aug 22;55(17):7199-204.
N'-Nitrosonornicotine (NNN) is one of the most abundant strong carcinogens in unburned tobacco and cigarette smoke and is classified by the International Agency for Research on Cancer as carcinogenic to humans. Human exposure to NNN mainly occurs upon use of tobacco products. It is also possible that additional amounts of NNN are formed endogenously. The goal of this study was to evaluate the inhibitory effect of some antioxidants, including ascorbic acid and grape seed extract (GSE), on endogenous NNN formation in rats treated with nornicotine and sodium nitrite by gavage twice daily for 3 days. The study included four groups of rats: (1) negative control group A, to which no chemical was administered; (2) negative control group B, treated with nornicotine alone (2.5 micromol per gavage); (3) positive control group, to which both nornicotine (2.5 micromol per gavage) and sodium nitrite (7.5 micromol per gavage) were administered; and (4) rats treated with nornicotine (2.5 micromol per gavage), inhibitor (7.5 or 37.5 micromol per gavage), and sodium nitrite (7.5 micromol per gavage). The mean (+/-SD) total amount of NNN in the 3-day urine of rats treated with both nornicotine and sodium nitrite was 4.78 +/- 2.88 nmol. The order of inhibition of endogenous NNN formation in rats at the molar ratio [nitrite]:[inhibitor] 1:5 was as follows: ascorbic acid (91%) > Dihydroxyfumaric acid (86%) approximately catechin (85%) > resveratrol (no inhibition). Treatment of rats with grape seed extract did not produce statistically significant inhibition of endogenous nornicotine nitrosation. This is the first study that demonstrates endogenous NNN formation in rats treated with nornicotine and sodium nitrite and effective inhibition of this process by ascorbic acid, dixydroxyfumaric acid, and catechin.
Effective attenuation of acute lung injury in vivo and the formyl peptide-induced neutrophil activation in vitro by CYL-26z through the phosphoinositide 3-kinase gamma pathway.[Pubmed:16887102]
Biochem Pharmacol. 2006 Sep 14;72(6):749-60.
5-[4-Acridin-9-ylamino]phenyl]-5-methyl-3-methylenedihydrofuran-2-one (CYL-26z) inhibited the polymorphonuclear leukocyte (PMNL) infiltration and protein leakage into the lungs in lipopolysaccharide (LPS)-induced acute lung injury (ALI) in mice as determined on the basis of PMNL and protein contents in bronchoalveolar lavage (BAL) fluid and myeloperoxidase (MPO) content in whole lung extracts. CYL-26z also attenuated the formyl-Met-Leu-Phe (fMLP)-induced neutrophil chemotaxis and respiratory burst in vitro (IC(50) 8.4+/-0.9microM and 2.0+/-0.6microM, respectively). CYL-26z had no effect on superoxide anion (O(2)(-)) generation during Dihydroxyfumaric acid autoxidation or on the NADPH oxidase activity in two cell-free systems (the arachidonic acid-induced assembly of NADPH oxidase and the preassembled oxidase caused by phorbol ester treatment), whereas it inhibited NaF-induced respiratory burst. Inhibition of respiratory burst by CYL-26z was readily reversible by washing. Only slight, but significant, inhibition of extracellular signal regulated kinase (ERK) phosphorylation and p38 mitogen-activated protein kinase (MAPK) activation in response to fMLP by CYL-26z up to 30microM was obtained. CYL-26z effectively blocked the formation of phosphatidylinositol-3,4,5-trisphosphate (PtdIns(3,4,5)P(3)) as determined by immunofluorescence microscopy and flow cytometry assays and the dual phosphorylation of protein kinase B (PKB/Akt) on S473 and T308 residues in fMLP-stimulated neutrophils. The membrane recruitment of p110gamma and Ras, the Ras activation, and the association between p110gamma and Ras were also attenuated by CYL-26z. These results indicate that the blockade of Ras activation by CYL-26z attenuated the downstream phosphoinositide 3-kinase (PI3K) gamma signaling, which is involved in chemoattractant-induced neutrophil chemotaxis and respiratory burst, and may have a beneficial anti-inflammatory effect on ALI.