QuinocetoneCAS# 81810-66-4 |
Quality Control & MSDS
3D structure
Package In Stock
Number of papers citing our products
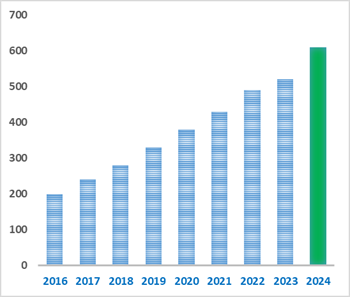
Cas No. | 81810-66-4 | SDF | Download SDF |
PubChem ID | 5387181 | Appearance | Powder |
Formula | C18H14N2O3 | M.Wt | 306.3 |
Type of Compound | N/A | Storage | Desiccate at -20°C |
Solubility | Soluble in Chloroform,Dichloromethane,Ethyl Acetate,DMSO,Acetone,etc. | ||
Chemical Name | (E)-1-(3-methyl-4-oxido-1-oxoquinoxalin-1-ium-2-yl)-3-phenylprop-2-en-1-one | ||
SMILES | CC1=C([N+](=O)C2=CC=CC=C2N1[O-])C(=O)C=CC3=CC=CC=C3 | ||
Standard InChIKey | IOKWXGMNRWVQHX-VAWYXSNFSA-N | ||
Standard InChI | InChI=1S/C18H14N2O3/c1-13-18(17(21)12-11-14-7-3-2-4-8-14)20(23)16-10-6-5-9-15(16)19(13)22/h2-12H,1H3/b12-11+ | ||
General tips | For obtaining a higher solubility , please warm the tube at 37 ℃ and shake it in the ultrasonic bath for a while.Stock solution can be stored below -20℃ for several months. We recommend that you prepare and use the solution on the same day. However, if the test schedule requires, the stock solutions can be prepared in advance, and the stock solution must be sealed and stored below -20℃. In general, the stock solution can be kept for several months. Before use, we recommend that you leave the vial at room temperature for at least an hour before opening it. |
||
About Packaging | 1. The packaging of the product may be reversed during transportation, cause the high purity compounds to adhere to the neck or cap of the vial.Take the vail out of its packaging and shake gently until the compounds fall to the bottom of the vial. 2. For liquid products, please centrifuge at 500xg to gather the liquid to the bottom of the vial. 3. Try to avoid loss or contamination during the experiment. |
||
Shipping Condition | Packaging according to customer requirements(5mg, 10mg, 20mg and more). Ship via FedEx, DHL, UPS, EMS or other couriers with RT, or blue ice upon request. |

Quinocetone Dilution Calculator

Quinocetone Molarity Calculator
1 mg | 5 mg | 10 mg | 20 mg | 25 mg | |
1 mM | 3.2648 mL | 16.3239 mL | 32.6477 mL | 65.2955 mL | 81.6193 mL |
5 mM | 0.653 mL | 3.2648 mL | 6.5295 mL | 13.0591 mL | 16.3239 mL |
10 mM | 0.3265 mL | 1.6324 mL | 3.2648 mL | 6.5295 mL | 8.1619 mL |
50 mM | 0.0653 mL | 0.3265 mL | 0.653 mL | 1.3059 mL | 1.6324 mL |
100 mM | 0.0326 mL | 0.1632 mL | 0.3265 mL | 0.653 mL | 0.8162 mL |
* Note: If you are in the process of experiment, it's necessary to make the dilution ratios of the samples. The dilution data above is only for reference. Normally, it's can get a better solubility within lower of Concentrations. |
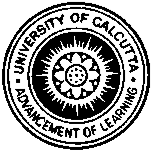
Calcutta University
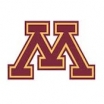
University of Minnesota
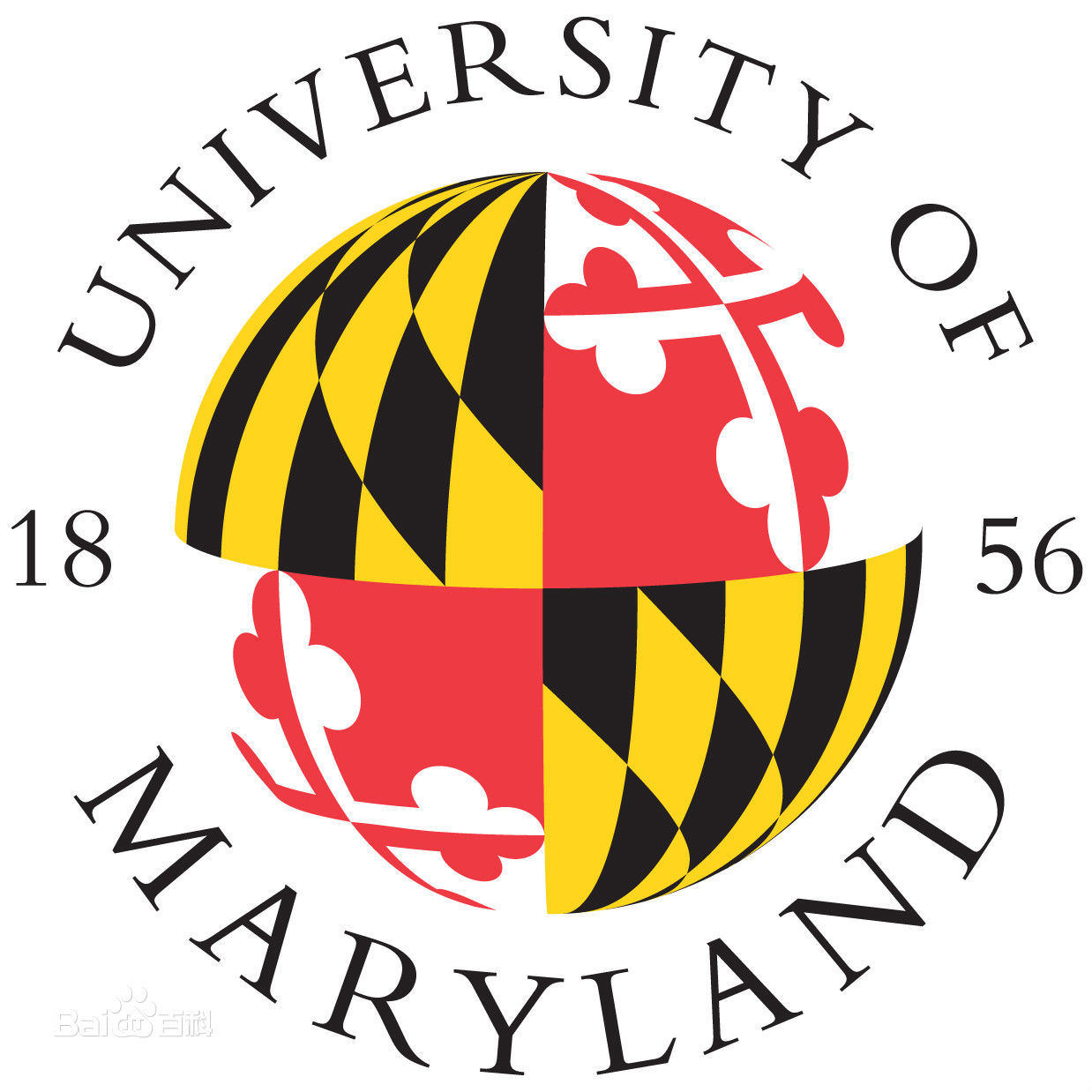
University of Maryland School of Medicine
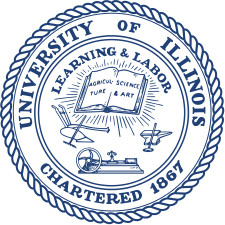
University of Illinois at Chicago
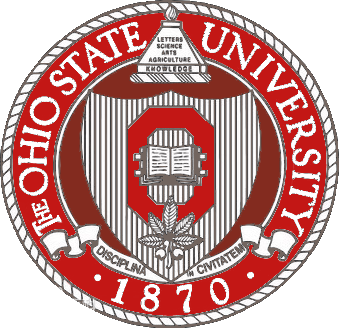
The Ohio State University
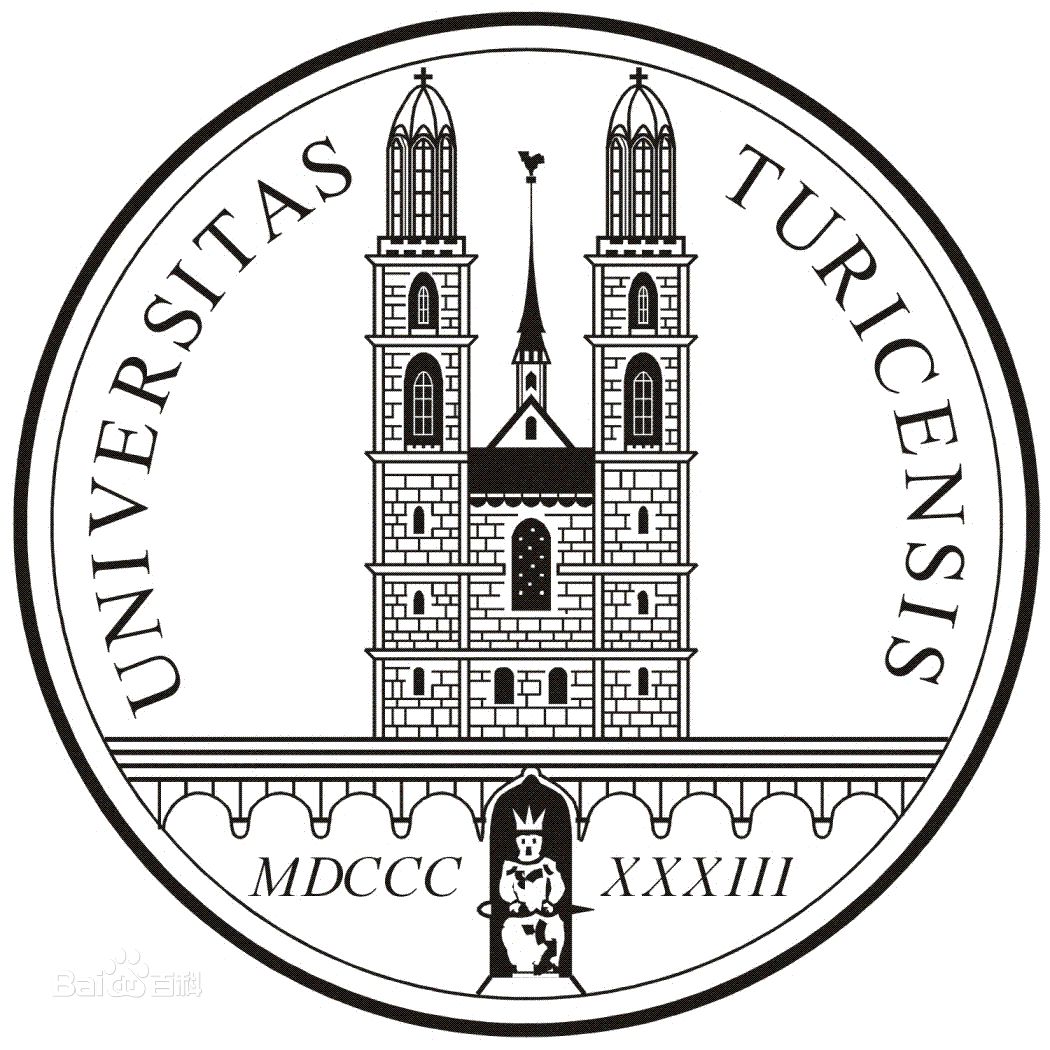
University of Zurich
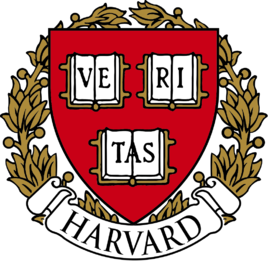
Harvard University
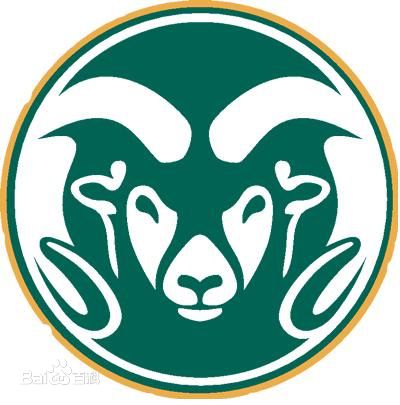
Colorado State University
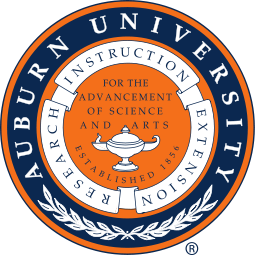
Auburn University
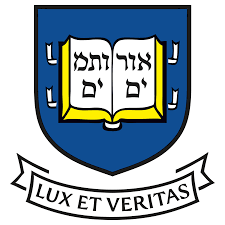
Yale University
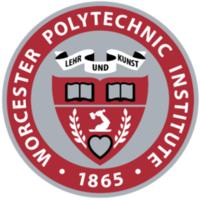
Worcester Polytechnic Institute
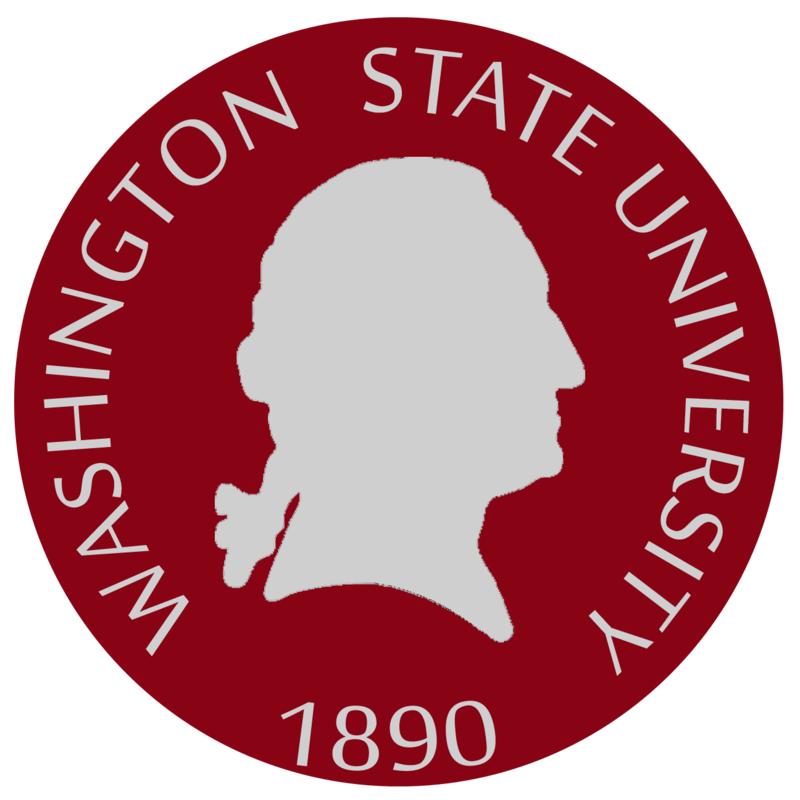
Washington State University
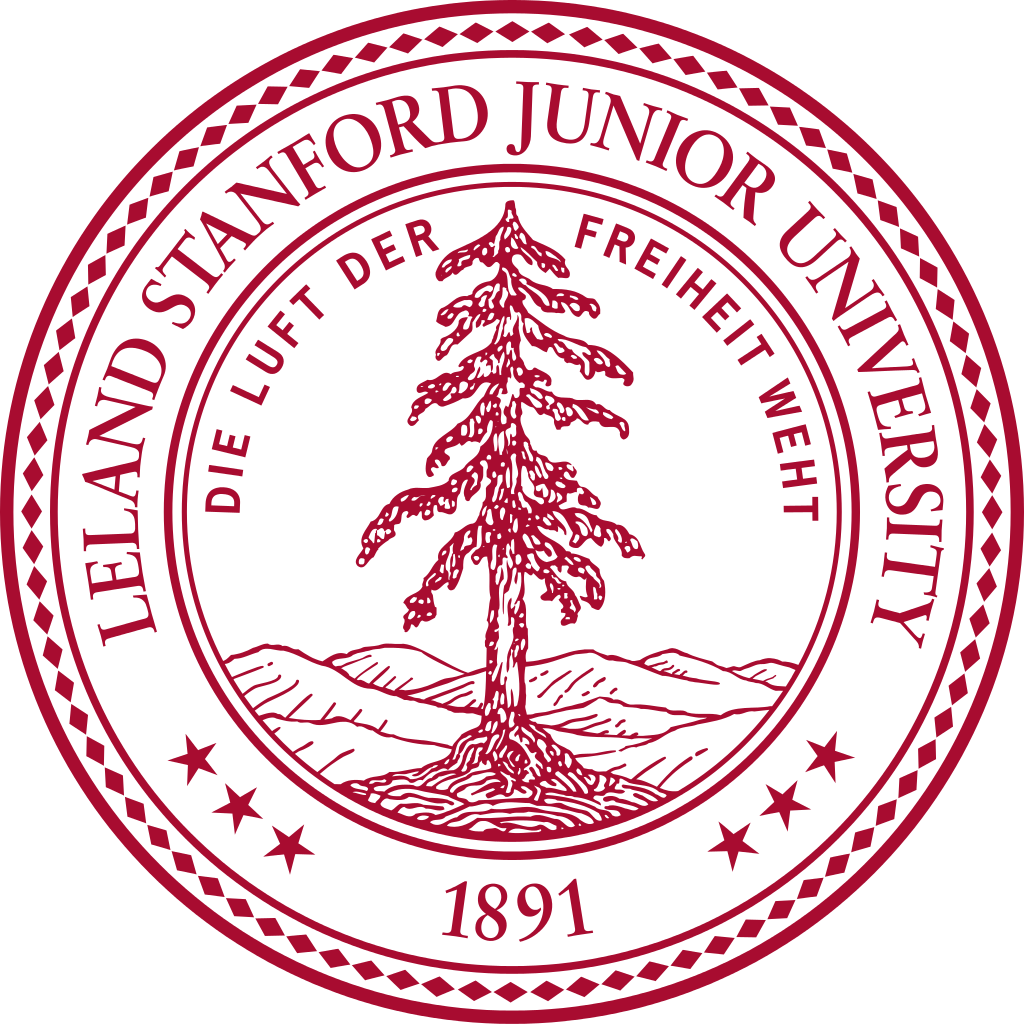
Stanford University
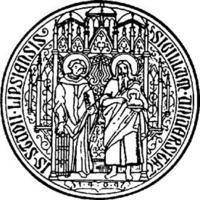
University of Leipzig
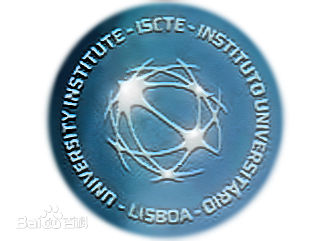
Universidade da Beira Interior
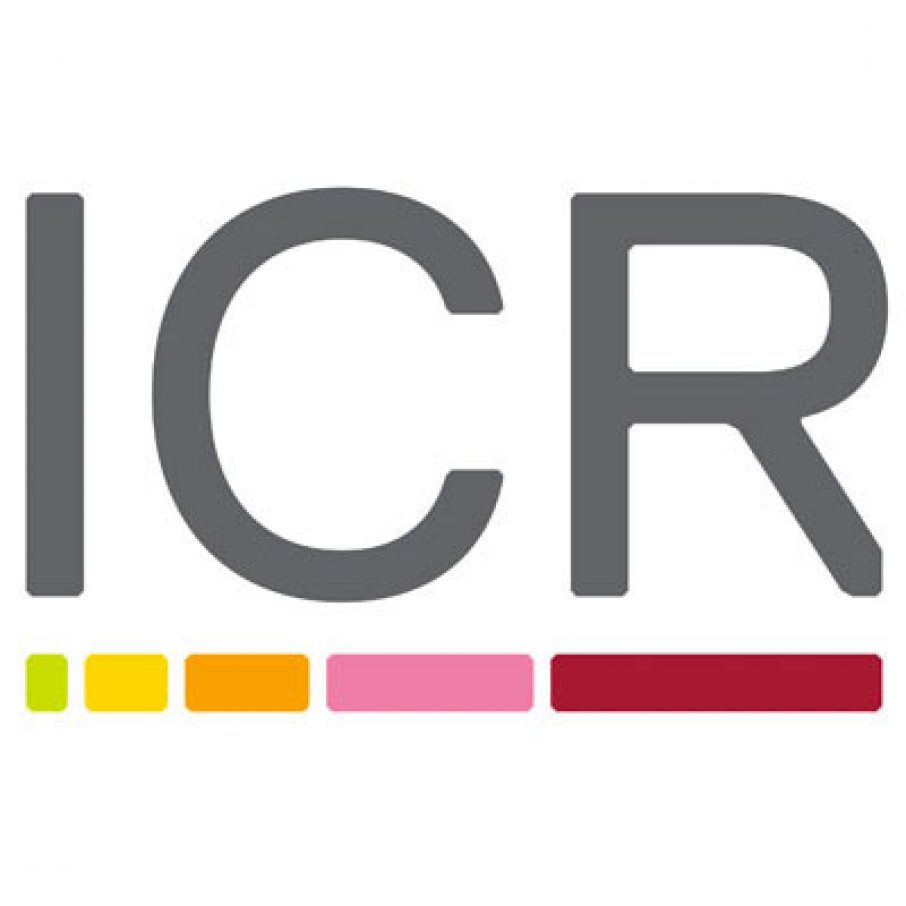
The Institute of Cancer Research
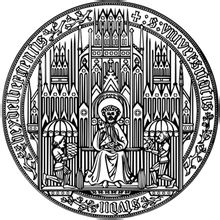
Heidelberg University
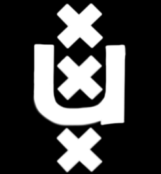
University of Amsterdam
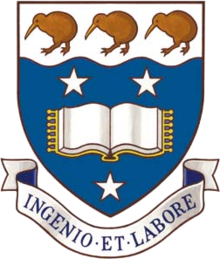
University of Auckland
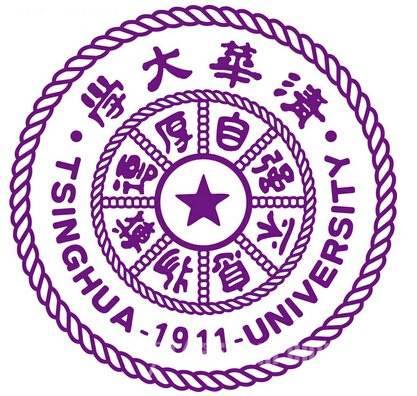
TsingHua University
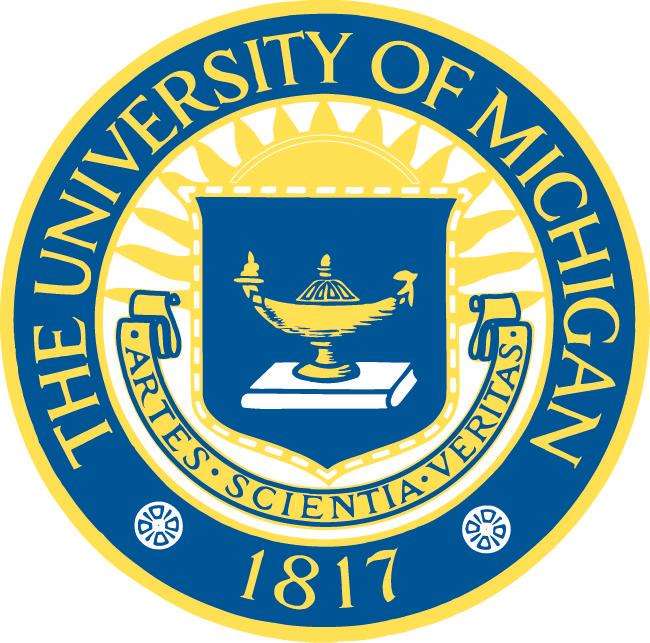
The University of Michigan
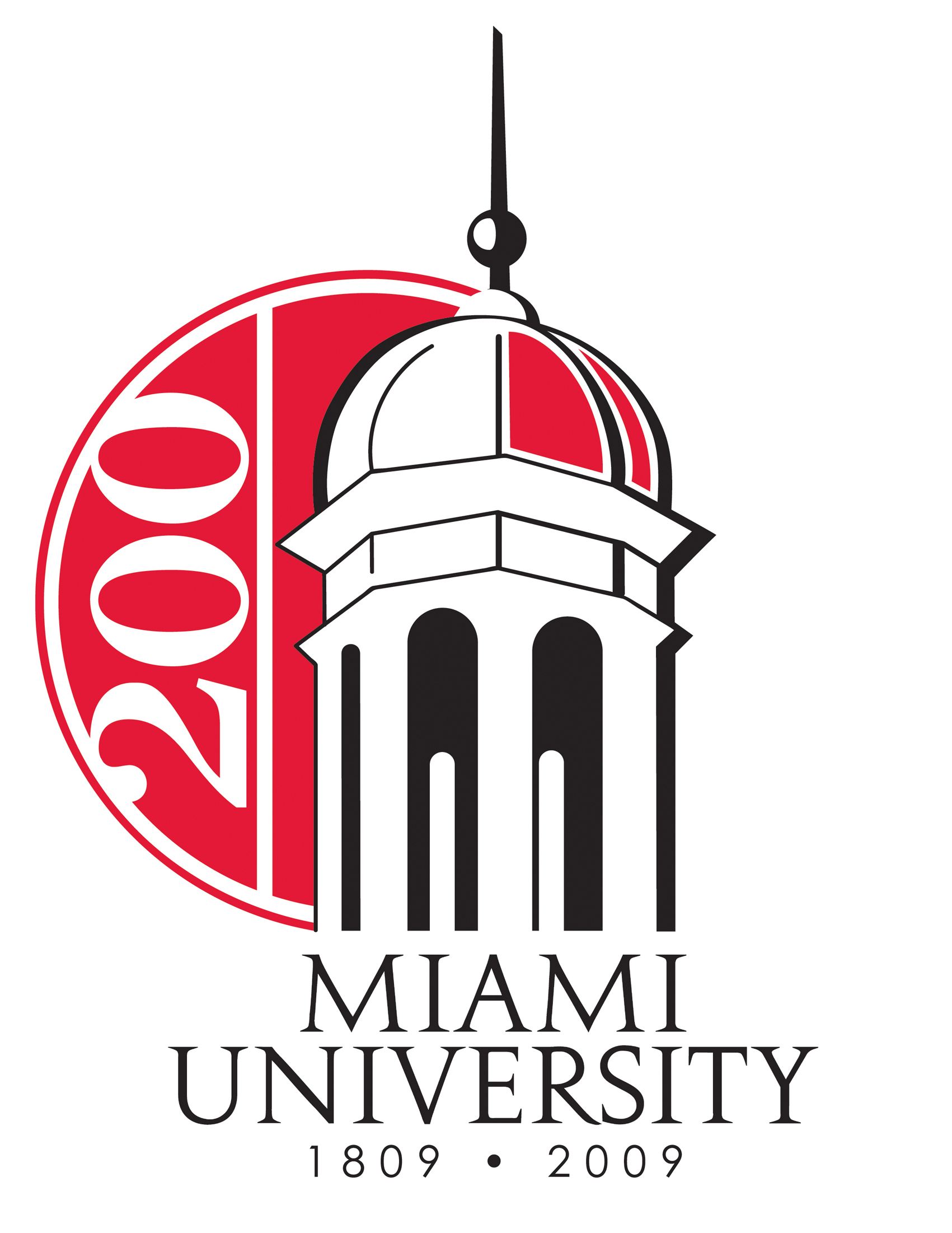
Miami University
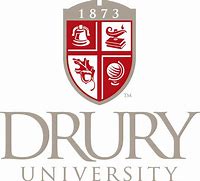
DRURY University
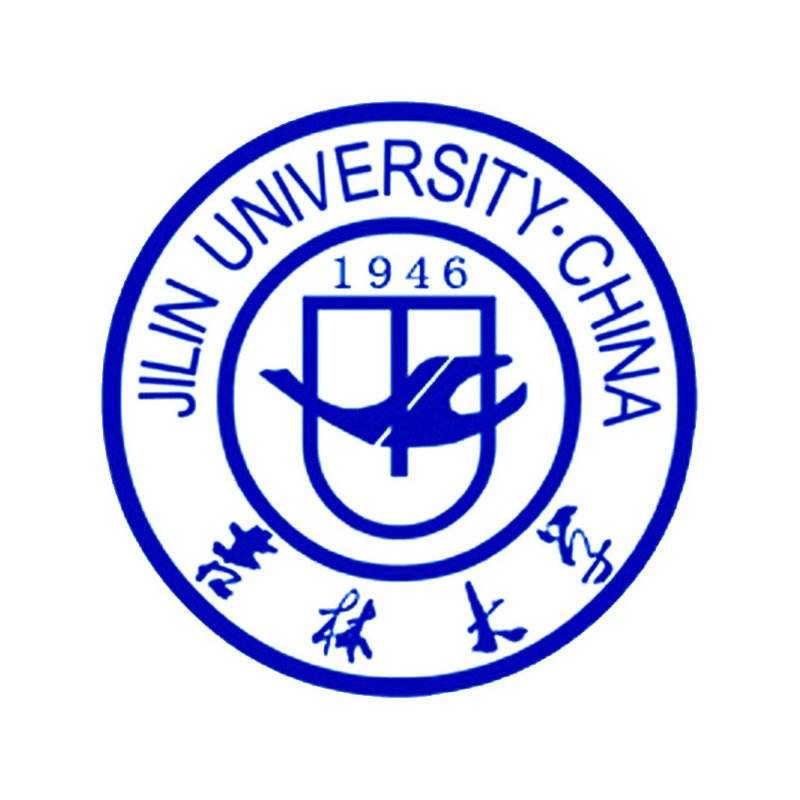
Jilin University
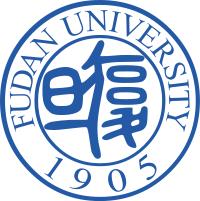
Fudan University
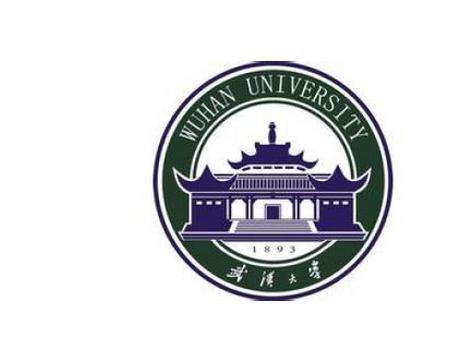
Wuhan University
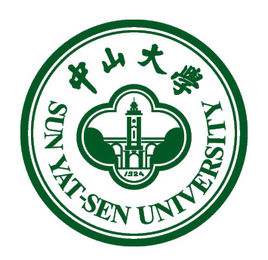
Sun Yat-sen University
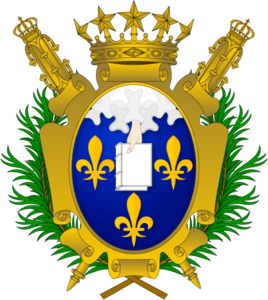
Universite de Paris
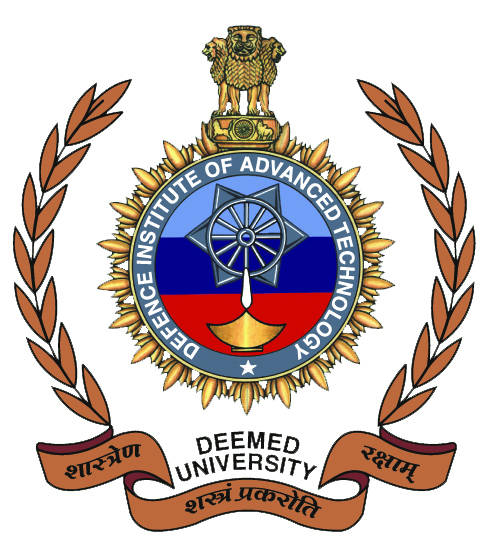
Deemed University
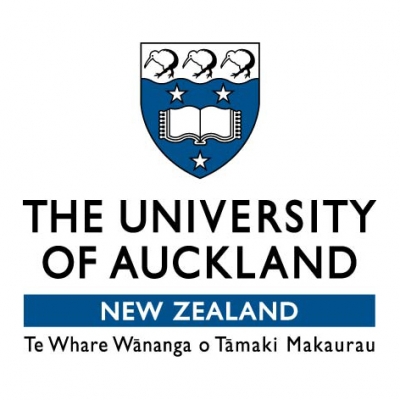
Auckland University
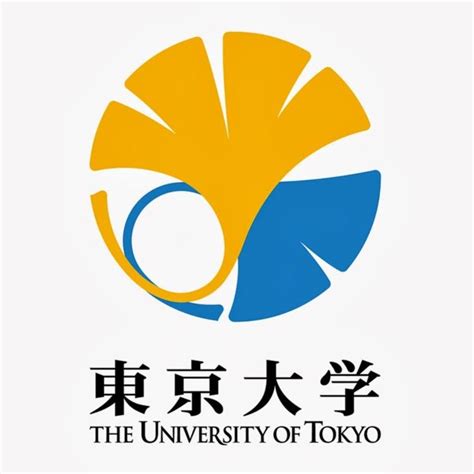
The University of Tokyo
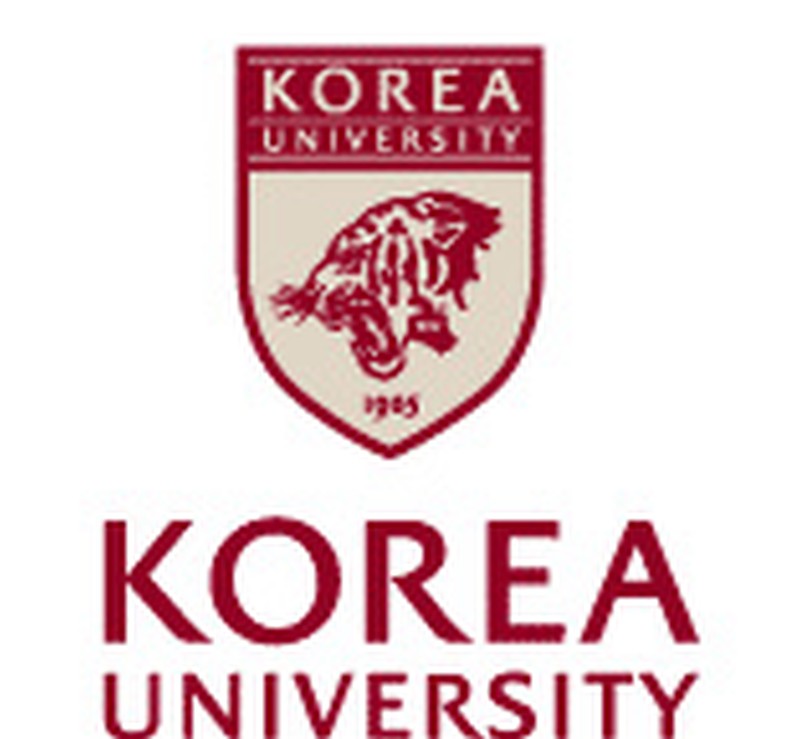
Korea University
- Methimepip dihydrobromide
Catalog No.:BCC7524
CAS No.:817636-54-7
- Praeruptorin B
Catalog No.:BCN4988
CAS No.:81740-07-0
- Bambuterol
Catalog No.:BCC5431
CAS No.:81732-65-2
- PSI-6130
Catalog No.:BCC1870
CAS No.:817204-33-4
- Rhmannioside D
Catalog No.:BCN2362
CAS No.:81720-08-3
- Rehmannioside C
Catalog No.:BCN8183
CAS No.:81720-07-2
- Rehmannioside B
Catalog No.:BCN8468
CAS No.:81720-06-1
- Rehmannioside A
Catalog No.:BCN2885
CAS No.:81720-05-0
- 18-Beta-hydroxy-3-epi-alpha-yohimbine
Catalog No.:BCN3518
CAS No.:81703-06-2
- 3-Dehydro-15-deoxoeucosterol
Catalog No.:BCN4351
CAS No.:81678-46-8
- Canusesnol A
Catalog No.:BCN4350
CAS No.:816456-90-3
- Withaperuvin C
Catalog No.:BCN6727
CAS No.:81644-34-0
- Neotriptophenolide
Catalog No.:BCN8059
CAS No.:81827-74-9
- Seneciocannabine
Catalog No.:BCN2128
CAS No.:81855-31-4
- Forskolin J
Catalog No.:BCN4352
CAS No.:81873-08-7
- Ganoderic acid B
Catalog No.:BCN3034
CAS No.:81907-61-1
- Ganoderic acid A
Catalog No.:BCN3033
CAS No.:81907-62-2
- 5,19-Epoxy-25-methoxycucurbita-6,23-dien-3-ol
Catalog No.:BCN1341
CAS No.:81910-39-6
- Momordicoside I aglycone
Catalog No.:BCN4353
CAS No.:81910-41-0
- Polyphyllin H
Catalog No.:BCN2834
CAS No.:81917-50-2
- Zofenopril calcium
Catalog No.:BCC5229
CAS No.:81938-43-4
- Z-Ligustilide
Catalog No.:BCC9193
CAS No.:81944-09-4
- 4(15),5,10(14)-Germacratrien-1-ol
Catalog No.:BCN4354
CAS No.:81968-62-9
- Splendoside
Catalog No.:BCN6647
CAS No.:81969-41-7
ROS-mediated oligomerization of VDAC2 is associated with quinocetone-induced apoptotic cell death.[Pubmed:29229420]
Toxicol In Vitro. 2018 Mar;47:195-206.
Quinocetone (QCT) has been approved and widely used as an animal feed additive in China since 2003. However, investigations indicate that QCT shows potential toxicity both in vitro and in vivo. Although voltage dependent anion channel 1 (VDAC1) involved in regulating QCT-induced apoptotic cell death has been established, the role of voltage dependent anion channel 2 (VDAC2) in QCT-induced toxicity remains unclear. In this study, we showed that QCT-induced cell death was coupled to VDAC2 oligomerization. Moreover, VDAC inhibitor 4, 4'-diisothiocyano stilbene-2, 2'-disulfonic acid (DIDS) alleviated QCT-induced cell death and VDAC2 oligomerization. Meanwhile, overexpression VDAC2 aggravated QCT-induced VDAC2 oligomerization. In addition, caspase inhibitor Z-VAD-FMK and reactive oxidative species (ROS) scavenger N-acetyl-l-cysteine (NAC) apparently blocked QCT-induced cell death and VDAC2 oligomerization. Finally, overexpression N-terminal truncated VDAC2 attenuated QCT-induced VDAC2 oligomerization but had no influence on its localization to mitochondria when comparing to the full length of VDAC2. Taken together, our results reveal that ROS-mediated VDAC2 oligomerization is associated with QCT-induced apoptotic cell death. The N-terminal region of VDAC2 is required for QCT-induced VDAC2 oligomerization.
Synthesis, In-Vitro Activity and Metabolic Properties of Quinocetone and Structurally Similar Compounds.[Pubmed:28979311]
Iran J Pharm Res. 2017 Spring;16(2):569-585.
To investigate the cytotoxicity mechanism of Quinocetone from the perspective of chemical structure, Quinocetone and other new quinoxaline-1, 4-dioxide derivatives were synthesized, and evaluated for their activities, and analysed for the metabolic characteristics. Quinocetone and other new quinoxaline-1,4-dioxide derivatives were synthesized, and evaluated for their activities, and analysed for the metabolic characteristics. The synthetic route started from 2-nitroaniline which was reacted with 3-bromopropanoic acid followed by the reaction of acetylacetone to afford 2-acetyl-3-methylquinoxaline-1, 4-dioxide. The aldol condensation of the later compound with aromatic aldehydes led to the formation of the Quinocetone structure similar compounds. A number of prepared derivatives exerted antimicrobial activities and cytotoxicity potency. Analysis of metabolic pathways in vitro displayed 2-propenyl and N-->O groups were the major sites. The results suggested 2-propenyl group exert important role in cytotoxicity of Quinocetone and is another major toxiccophore for Quinocetone, and different electronic substituents in arylidene aryl ring could affect the electronic arrangement of 2-propenyl and N-->O groups to chang the cytostatic potency.
Plasma pharmacokinetics of quinocetone in ducks after oral and intravenous administration.[Pubmed:28730638]
J Vet Pharmacol Ther. 2018 Feb;41(1):142-147.
Quinocetone (QCT), an antimicrobial growth promoter, is widely used in food-producing animals. However, information about pharmacokinetics (PK) of QCT in ducks still remains unavailable up to now. In this study, QCT and its major metabolites (1-desoxyQuinocetone, di-desoxyQuinocetone and 3-methyl-quinoxaline-2-carboxylic) in ducks were studied using a simple and sensitive UHPLC-MS/MS assay. Twenty ducks were divided into two groups. (n = 10/group). One group received QCT by oral administration at dose of 40 mg/kg while another group received QCT intravenously at 10 mg/kg. Plasma samples were collected at various time points from 0 to 96 hr. QCT and its major metabolites in duck plasma samples were extracted by 1 ml acetonitrile and detected by UHPLC-MS/MS, with the gradient mobile phase that consisted of 0.1% formic acid in water (A) and acetonitrile (B). A noncompartment analysis was used to calculate the PK parameters. The results showed that following oral dosing, the peak plasma concentration (Cmax ) of QCT was 32.14 ng/ml and the area under the curve (AUCINF_obs) was 233.63 (h ng)/ ml. Following intravenous dosing, the Cmax , AUCINF_obs and Vss_obs were 96.70 ng/ml, 152.34 (h ng)/ ml and 807.00 L/kg, respectively. These data indicated that the QCT was less absorbed in vivo following oral administration, with low bioavailability (38.43%). QCT and its major metabolites such as 1-desoxyQuinocetone and 3-methyl-quinoxaline-2-carboxylic were detected at individual time points in individual ducks, while the di-desoxyQuinocetone was not detected in all time points in all ducks. This study enriches basic scientific data about pharmacokinetics of QCT in ducks after oral and intravenous administration and will be beneficial for clinical application in ducks.
Quinocetone induces mitochondrial apoptosis in HepG2 cells through ROS-dependent promotion of VDAC1 oligomerization and suppression of Wnt1/beta-catenin signaling pathway.[Pubmed:28343033]
Food Chem Toxicol. 2017 Jul;105:161-176.
Quinocetone (QCT) has been used as an animal feed additive in China since 2003. However, investigations indicate that QCT has potential toxicity due to the fact that it shows cytotoxicity, genotoxicity, hepatotoxicity, nephrotoxicity and immunotoxicity in vitro and animal models. Although QCT-induced mitochondrial apoptosis has been established, the molecular mechanism remains unclear. This study was aimed to investigate the role of voltage-dependent anion channel 1 (VDAC1) oligomerization and Wnt/beta-catenin pathway in QCT-induced mitochondrial apoptosis. The results showed VDAC inhibitor 4, 4-diisothiocyano stilbene-2, 2-disulfonic acid (DIDS) partly compromised QCT-induced cell viability decrease (from 34.1% to 68.5%) and mitochondrial apoptosis accompanied by abating VDAC1 oligomerization, cytochrome c (Cyt c) release and the expression levels of cleaved caspase-9, -3 and poly (ADP-ribose) polymerase (PARP). Meanwhile, overexpression VDAC1 exacerbated QCT-induced VDAC1 oligomerization and Cyt c release. In addition, lithium chloride (LiCl), an activator of Wnt/beta-catenin pathway, markedly attenuated QCT-induced mitochondrial apoptosis by partly restoring the expression levels of Wnt1 and beta-catenin. Finally, reactive oxygen species (ROS) scavenger N-acetyl-l-cysteine (NAC) obviously blocked QCT-induced VDAC1 oligomerization and the inhibition of Wnt1/beta-catenin pathway. Taken together, our results reveal that QCT induces mitochondrial apoptosis by ROS-dependent promotion of VDAC1 oligomerization and suppression of Wnt1/beta-catenin pathway.
Physiologically based pharmacokinetic model for quinocetone in pigs and extrapolation to mequindox.[Pubmed:28001497]
Food Addit Contam Part A Chem Anal Control Expo Risk Assess. 2017 Feb;34(2):192-210.
Physiologically based pharmacokinetic (PBPK) models are scientific methods used to predict veterinary drug residues that may occur in food-producing animals, and which have powerful extrapolation ability. Quinocetone (QCT) and mequindox (MEQ) are widely used in China for the prevention of bacterial infections and promoting animal growth, but their abuse causes a potential threat to human health. In this study, a flow-limited PBPK model was developed to simulate simultaneously residue depletion of QCT and its marker residue dideoxyQuinocetone (DQCT) in pigs. The model included compartments for blood, liver, kidney, muscle and fat and an extra compartment representing the other tissues. Physiological parameters were obtained from the literature. Plasma protein binding rates, renal clearances and tissue/plasma partition coefficients were determined by in vitro and in vivo experiments. The model was calibrated and validated with several pharmacokinetic and residue-depletion datasets from the literature. Sensitivity analysis and Monte Carlo simulations were incorporated into the PBPK model to estimate individual variation of residual concentrations. The PBPK model for MEQ, the congener compound of QCT, was built through cross-compound extrapolation based on the model for QCT. The QCT model accurately predicted the concentrations of QCT and DQCT in various tissues at most time points, especially the later time points. Correlation coefficients between predicted and measured values for all tissues were greater than 0.9. Monte Carlo simulations showed excellent consistency between estimated concentration distributions and measured data points. The extrapolation model also showed good predictive power. The present models contribute to improve the residue monitoring systems of QCT and MEQ, and provide evidence of the usefulness of PBPK model extrapolation for the same kinds of compounds.
Further investigations into the genotoxicity of quinoxaline-di-N-oxides and their primary metabolites.[Pubmed:27170491]
Food Chem Toxicol. 2016 Jul;93:145-57.
Quinoxaline-di-N-oxides (QdNOs) are potential antibacterial agents with a wide range of biological properties. Quinocetone (QCT), carbadox (CBX), olaquindox (OLA), mequindox (MEQ) and cyadox (CYA) are classical QdNOs. Though the genotoxicity of parent drugs has been evaluated, the genotoxicity of their primary N --> O reduced metabolites remains unclear. In the present study, a battery of four different short-term tests, mouse lymphoma assay (MLA), Ames test, chromosomal aberration assay in vitro and bone marrow erythrocyte micronucleus assay in vivo was carried out to investigate the genotoxicity of the six primary N --> O reduced metabolites. Additionally, the genotoxicity of five parent drugs was evaluated by the MLA. Strong genotoxicity of N1-MEQ, B-MEQ and B-CBX was found in three of the assays but not in the Ames assay, and the rank order was N1-MEQ>B-MEQ>B-CBX that is consistent with prototype QdNOs. Negative results for the five QdNOs were noted in the MLA. We present for the first time a comparison of the genotoxicity of primary N --> O reduced metabolites, and evaluate the ability of five QdNOs to cause mutations in the MLA. The present study demonstrates that metabolites are involved in genetic toxicity mediated by QdNOs, and improve the prudent use of QdNOs for public health.
AKT/TSC2/p70S6K signaling pathway is involved in quinocetone-induced death-promoting autophagy in HepG2 cells.[Pubmed:27098396]
Toxicol Mech Methods. 2016 May;26(4):301-10.
Quinocetone (QCT, 3-methyl-2-quinoxalin benzenevinylketo-1, 4-dioxide) is widely used as a veterinary drug and animal feed additive in China. Although it promotes growth and improves feed efficiency, QCT's in vitro and in vivo toxicities remain uncertain. This study was conducted to explore the mechanism of QCT-induced autophagy in HepG2 cells. By the results obtained from monodansylcadaverine (MDC) staining, ultrastructural observation by transmission electron microscopy (TEM), as well as Western blotting analysis for LC3, p62, and Beclin-1, it was demonstrated that QCT induced autophagy in HepG2 cells. Furthermore, PI3K/AKT inhibitor significantly enhanced QCT-induced autophagy, while TSC2 knockdown attenuated this process. In addition, inhibition of autophagy by pharmacological approach remarkably increased the viability of QCT-treated cells detected by MTT assay, suggesting that QCT-triggered autophagy may play as a promotion mechanism for cell death. Meanwhile, apoptosis was markedly downregulated after autophagy blockage, and evaluated by flow cytometry and Western blotting analysis for caspase-3 cleavage. Consequently, these results suggested that QCT-induced autophagy was mediated by AKT/TSC2/p70S6K signaling pathway, and inhibition of autophagy promoted QCT-treated cell survival by attenuating apoptosis.
Quinocetone triggered ER stress-induced autophagy via ATF6/DAPK1-modulated mAtg9a trafficking.[Pubmed:27085326]
Cell Biol Toxicol. 2016 Apr;32(2):141-52.
The present study is undertaken to explore Quinocetone-induced autophagy and its possible mechanism. Western blotting and green fluorescence protein (GFP)-LC3 vector transfection were performed to determine the ratio of LC3 conversion and its subcellular localization. Results revealed that the Quinocetone induced autophagy in time- and dose-dependent manners. Besides, we tested the expressions of immunoglobulin heavy chain binding protein (BiP) and C/EBP homologous protein (CHOP) and the transcription of BiP, HerpUD, and sec24D by western blotting and RT-PCR, respectively. Results showed that Quinocetone also induced endoplasmic reticulum (ER) stress during Quinocetone-induced autophagy. Furthermore, we observed the cleavage of ATF6, the phosphorylation of MRLC, and the expression of death-associated protein kinase (DAPK1) by western blotting; the transcription of DAPK1 by RT-PCR; and the subcellular localization of ATF6 and mAtg9 by immunofluorescence. These results suggest that Quinocetone stimulates the MRLC-mediated mAtg9 trafficking, which is critical for autophagosome formation, via the ATF6 upregulated expression of DAPK1. Last, we generated ATF6 and DAPK1 stable knockdown HepG2 cell lines and found that the conversion ratios of LC3 were decreased upon the treatment of Quinocetone. Together, we propose that Quinocetone induces autophagy through ER stress signaling pathway-induced cytoskeleton activation.