PKA inhibitor fragment (6-22) amidePKA inhibitor CAS# 121932-06-7 |
- Trelagliptin succinate
Catalog No.:BCC2015
CAS No.:1029877-94-8
- NVP DPP 728 dihydrochloride
Catalog No.:BCC2365
CAS No.:247016-69-9
- Sitagliptin phosphate monohydrate
Catalog No.:BCC2111
CAS No.:654671-77-9
- Alogliptin (SYR-322)
Catalog No.:BCC2113
CAS No.:850649-61-5
- Trelagliptin
Catalog No.:BCC2014
CAS No.:865759-25-7
- DPPI 1c hydrochloride
Catalog No.:BCC2363
CAS No.:866396-34-1
Quality Control & MSDS
Number of papers citing our products
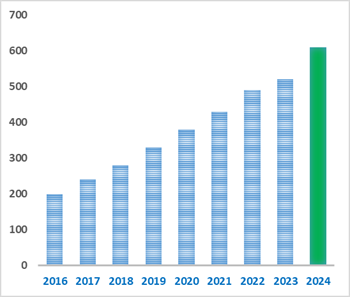
Chemical structure
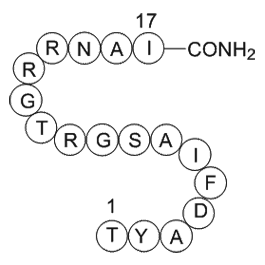
3D structure
Cas No. | 121932-06-7 | SDF | Download SDF |
PubChem ID | 16155227 | Appearance | Powder |
Formula | C80H130N28O24 | M.Wt | 1868.08 |
Type of Compound | N/A | Storage | Desiccate at -20°C |
Synonyms | Protein kinase inhibitor-(6-22)-amide, PKI-(6-22)-amide | ||
Solubility | Soluble to 1 mg/ml in water | ||
Sequence | TYADFIASGRTGRRNAI (Modifications: Ile-17 = C-terminal amide) | ||
SMILES | CCC(C)C(C(=O)N)NC(=O)C(C)NC(=O)C(CC(=O)N)NC(=O)C(CCCNC(=N)N)NC(=O)C(CCCNC(=N)N)NC(=O)CNC(=O)C(C(C)O)NC(=O)C(CCCNC(=N)N)NC(=O)CNC(=O)C(CO)NC(=O)C(C)NC(=O)C(C(C)CC)NC(=O)C(CC1=CC=CC=C1)NC(=O)C(CC(=O)O)NC(=O)C(C)NC(=O)C(CC2=CC=C(C=C2)O)NC(=O)C(C(C)O)N | ||
Standard InChIKey | VAKHFAFLRUNHLQ-PEBJKXEYSA-N | ||
Standard InChI | InChI=1S/C80H130N28O24/c1-10-37(3)60(63(83)118)106-66(121)41(7)96-72(127)52(32-55(81)113)103-69(124)49(22-17-29-92-80(88)89)100-68(123)47(20-15-27-90-78(84)85)98-57(115)35-94-76(131)62(43(9)111)108-70(125)48(21-16-28-91-79(86)87)99-56(114)34-93-67(122)54(36-109)105-65(120)40(6)97-77(132)61(38(4)11-2)107-74(129)51(30-44-18-13-12-14-19-44)102-73(128)53(33-58(116)117)101-64(119)39(5)95-71(126)50(104-75(130)59(82)42(8)110)31-45-23-25-46(112)26-24-45/h12-14,18-19,23-26,37-43,47-54,59-62,109-112H,10-11,15-17,20-22,27-36,82H2,1-9H3,(H2,81,113)(H2,83,118)(H,93,122)(H,94,131)(H,95,126)(H,96,127)(H,97,132)(H,98,115)(H,99,114)(H,100,123)(H,101,119)(H,102,128)(H,103,124)(H,104,130)(H,105,120)(H,106,121)(H,107,129)(H,108,125)(H,116,117)(H4,84,85,90)(H4,86,87,91)(H4,88,89,92)/t37-,38-,39-,40-,41-,42+,43+,47-,48-,49-,50-,51-,52-,53-,54-,59-,60-,61-,62-/m0/s1 | ||
General tips | For obtaining a higher solubility , please warm the tube at 37 ℃ and shake it in the ultrasonic bath for a while.Stock solution can be stored below -20℃ for several months. We recommend that you prepare and use the solution on the same day. However, if the test schedule requires, the stock solutions can be prepared in advance, and the stock solution must be sealed and stored below -20℃. In general, the stock solution can be kept for several months. Before use, we recommend that you leave the vial at room temperature for at least an hour before opening it. |
||
About Packaging | 1. The packaging of the product may be reversed during transportation, cause the high purity compounds to adhere to the neck or cap of the vial.Take the vail out of its packaging and shake gently until the compounds fall to the bottom of the vial. 2. For liquid products, please centrifuge at 500xg to gather the liquid to the bottom of the vial. 3. Try to avoid loss or contamination during the experiment. |
||
Shipping Condition | Packaging according to customer requirements(5mg, 10mg, 20mg and more). Ship via FedEx, DHL, UPS, EMS or other couriers with RT, or blue ice upon request. |
Description | Potent inhibitor of cAMP-dependent protein kinase (PKA) (Ki = 2.5 nM); derived from the active portion of the heat-stable PKA inhibitor protein PKI. |

PKA inhibitor fragment (6-22) amide Dilution Calculator

PKA inhibitor fragment (6-22) amide Molarity Calculator
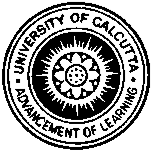
Calcutta University
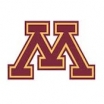
University of Minnesota
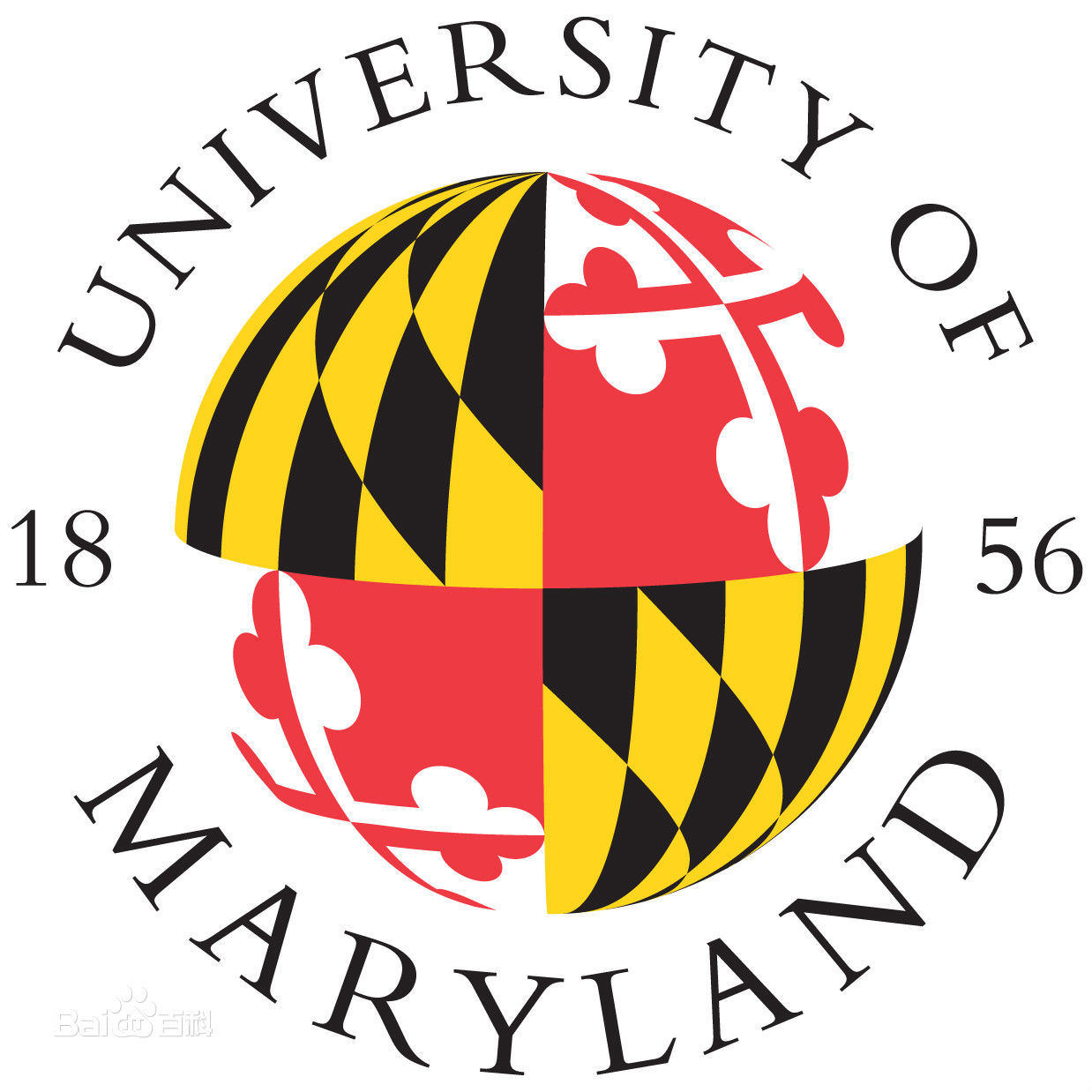
University of Maryland School of Medicine
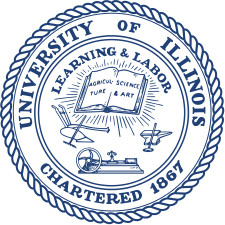
University of Illinois at Chicago
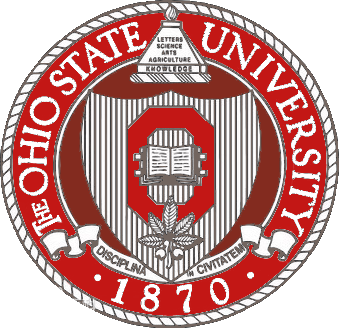
The Ohio State University
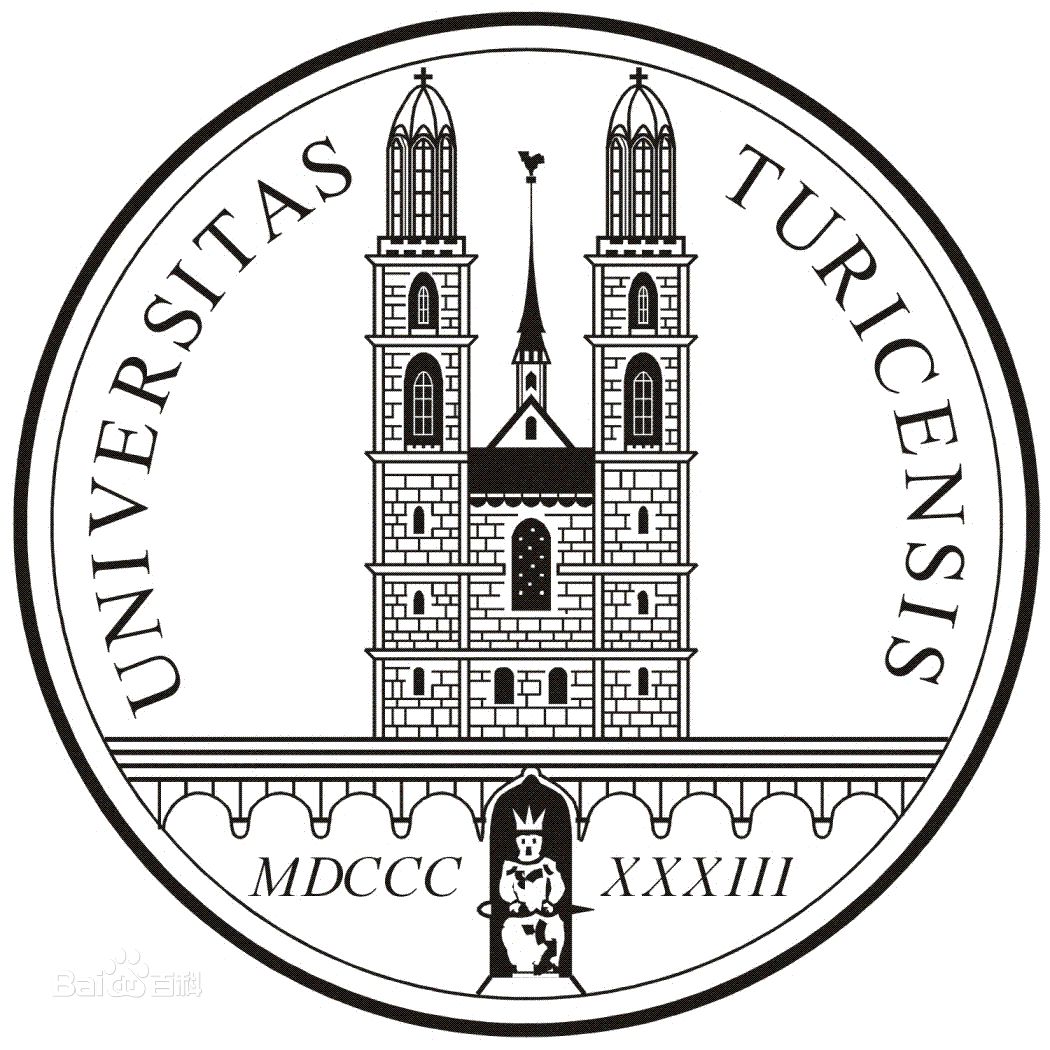
University of Zurich
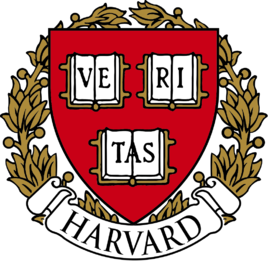
Harvard University
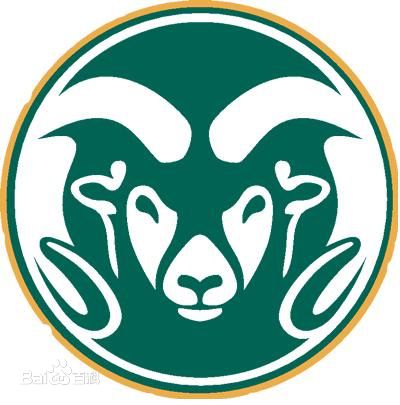
Colorado State University
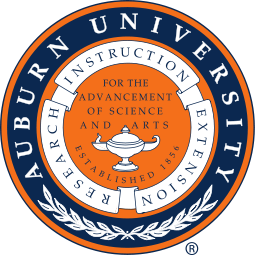
Auburn University
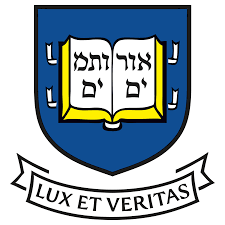
Yale University
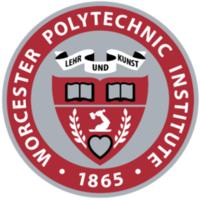
Worcester Polytechnic Institute
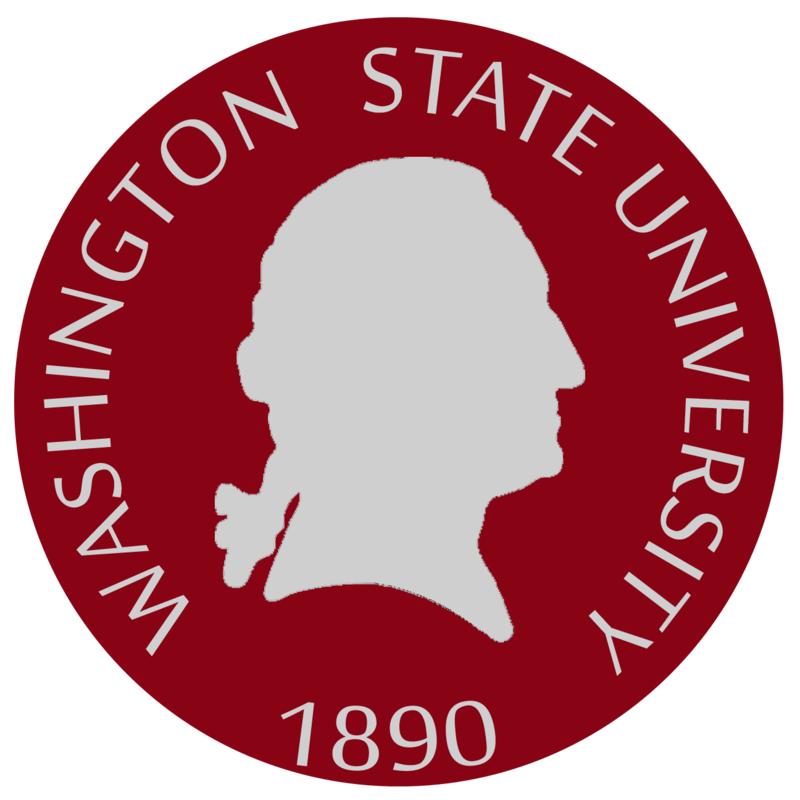
Washington State University
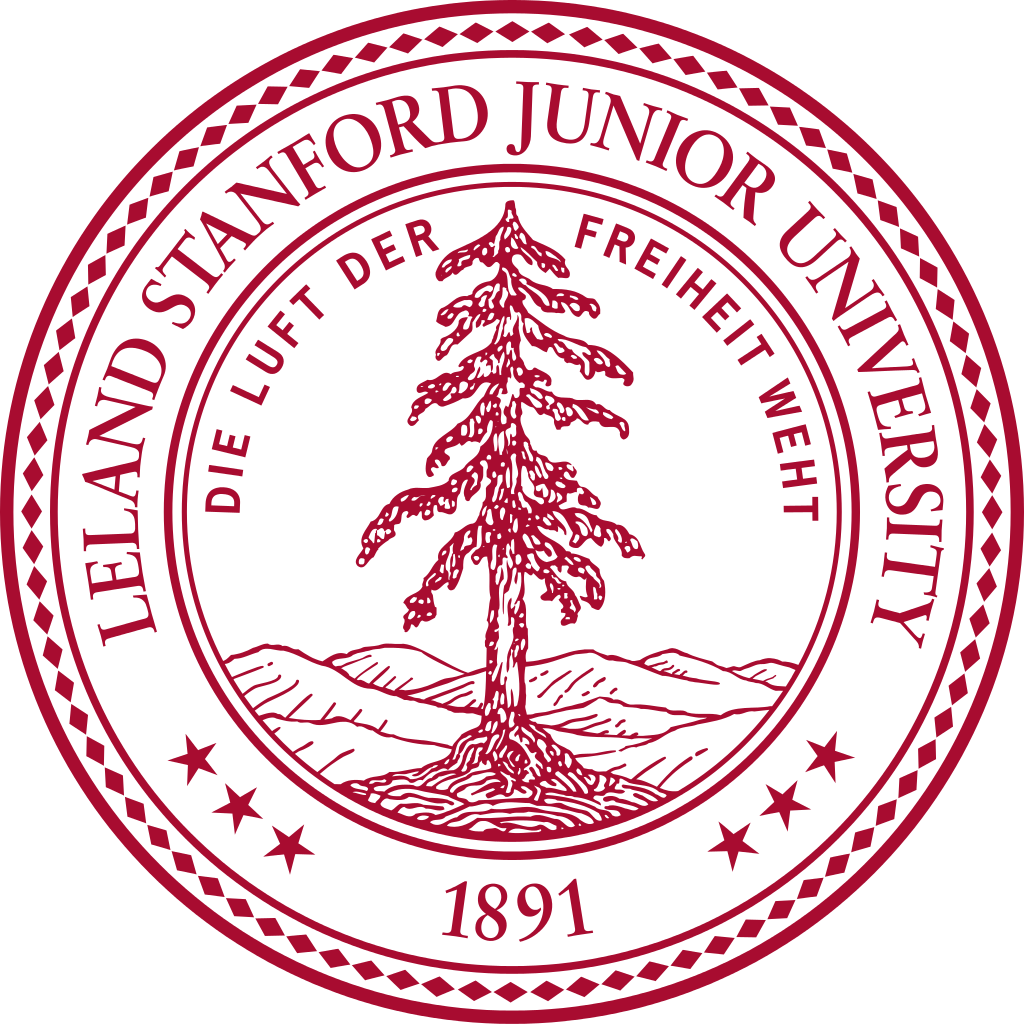
Stanford University
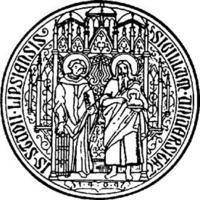
University of Leipzig
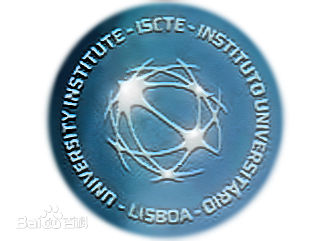
Universidade da Beira Interior
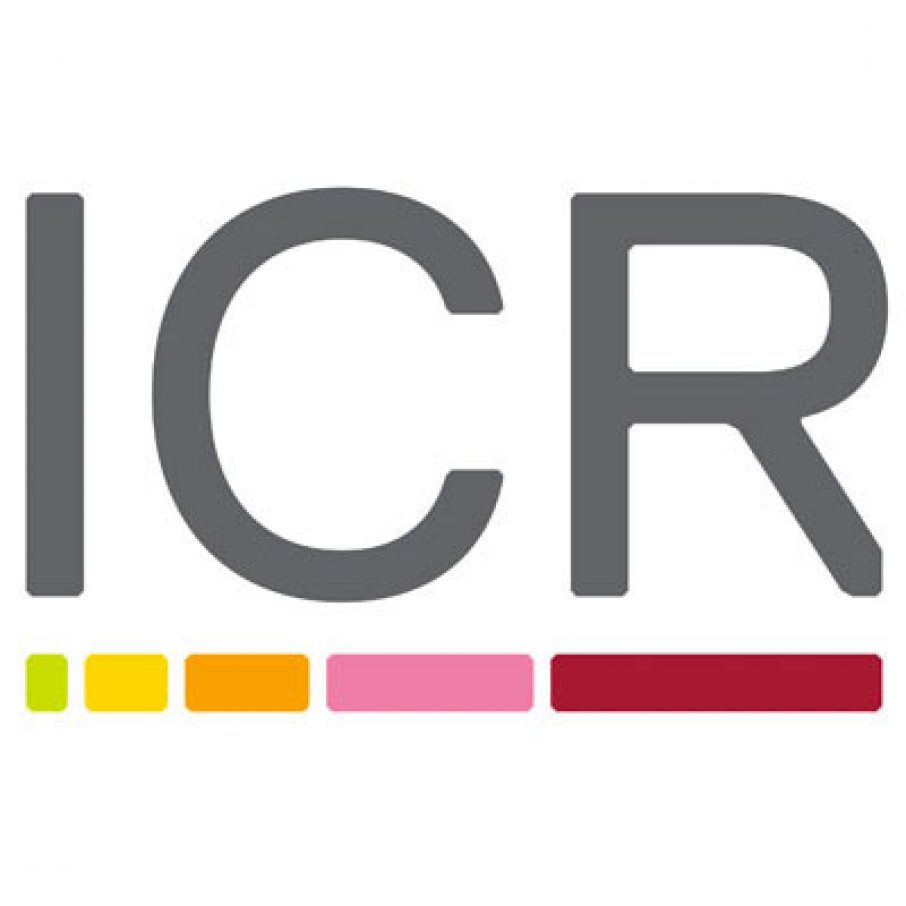
The Institute of Cancer Research
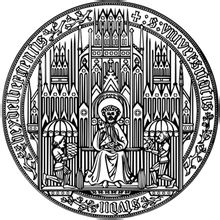
Heidelberg University
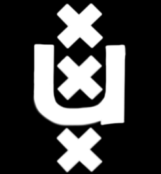
University of Amsterdam
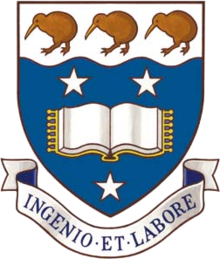
University of Auckland
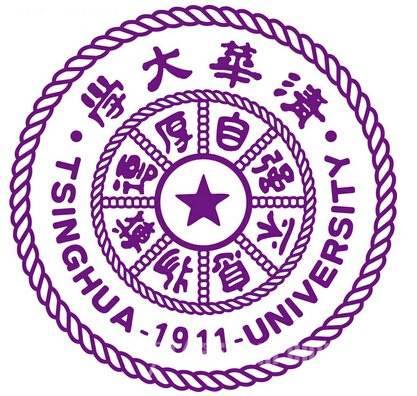
TsingHua University
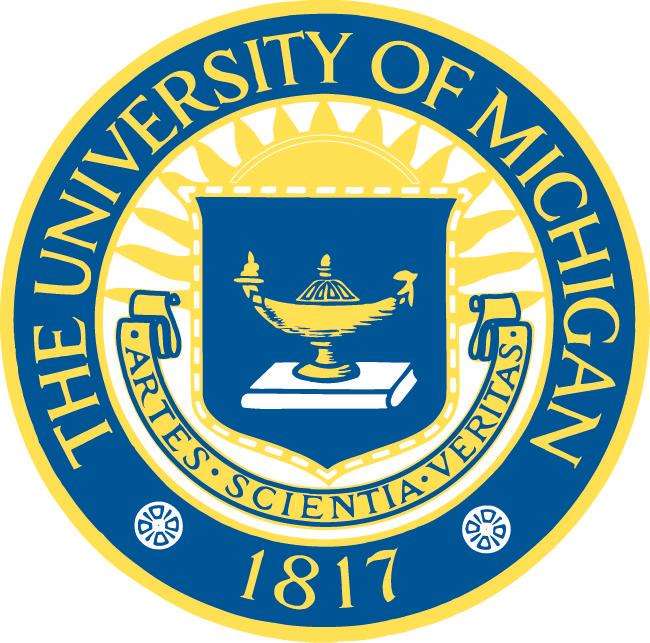
The University of Michigan
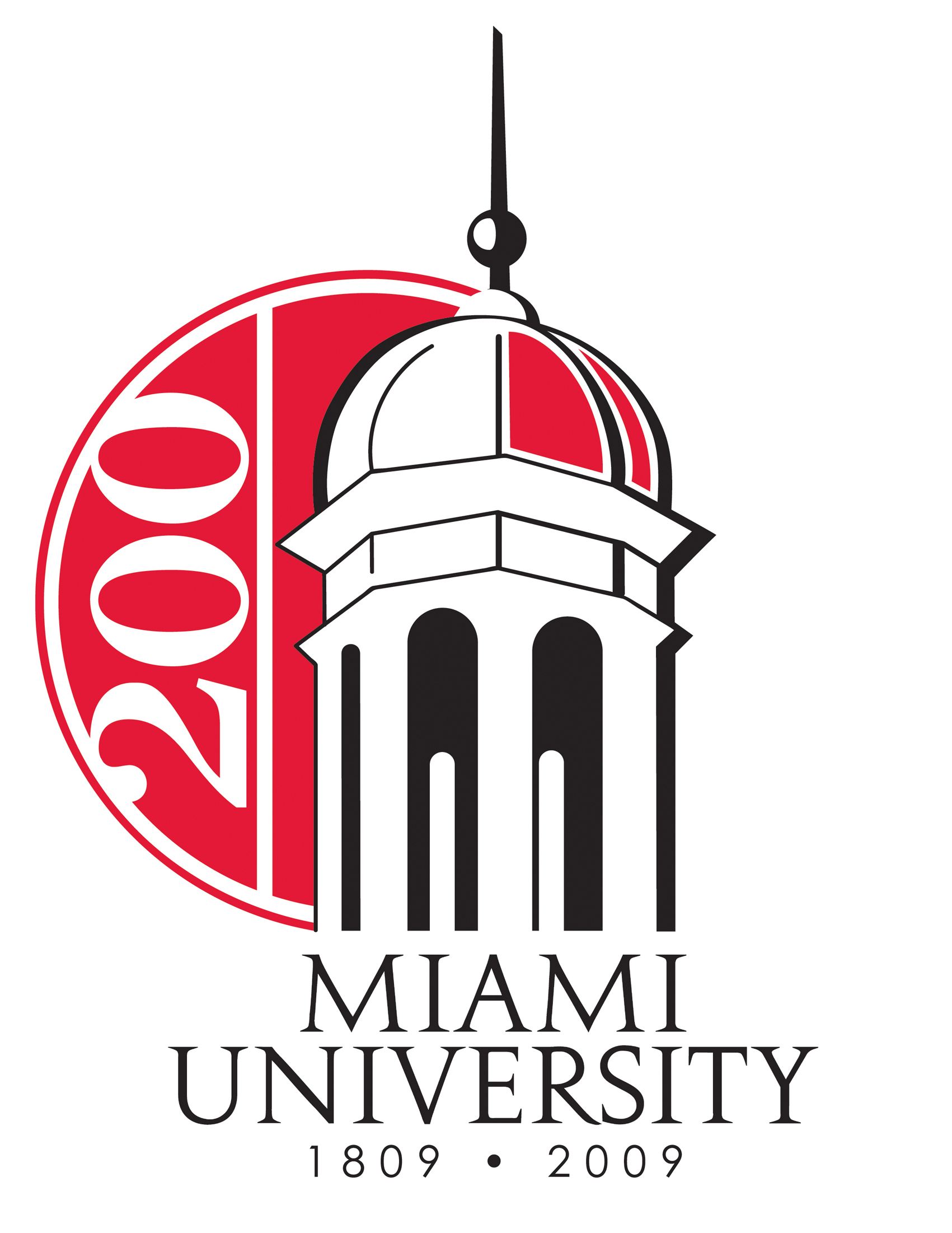
Miami University
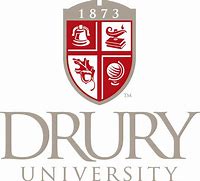
DRURY University
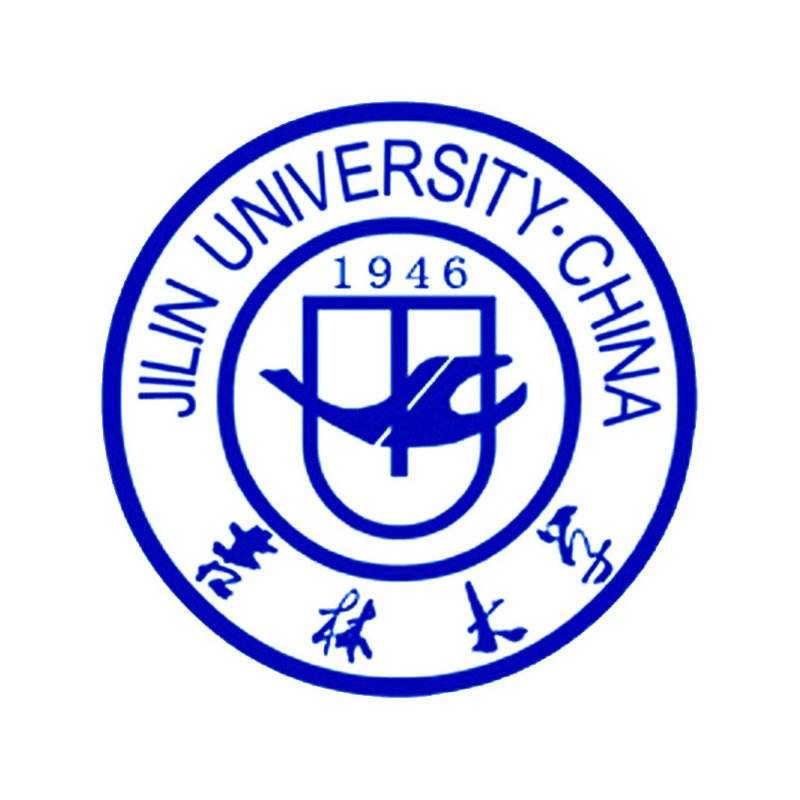
Jilin University
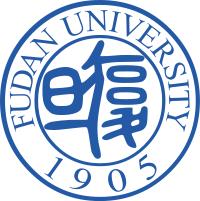
Fudan University
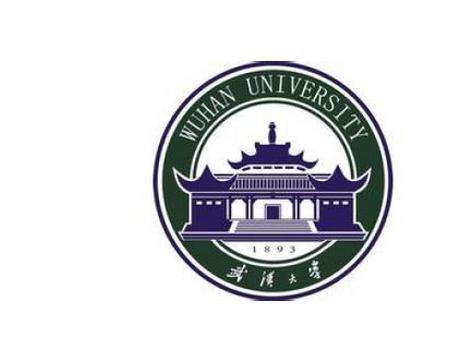
Wuhan University
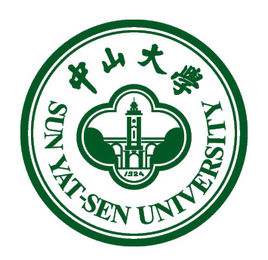
Sun Yat-sen University
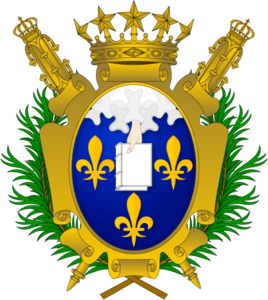
Universite de Paris
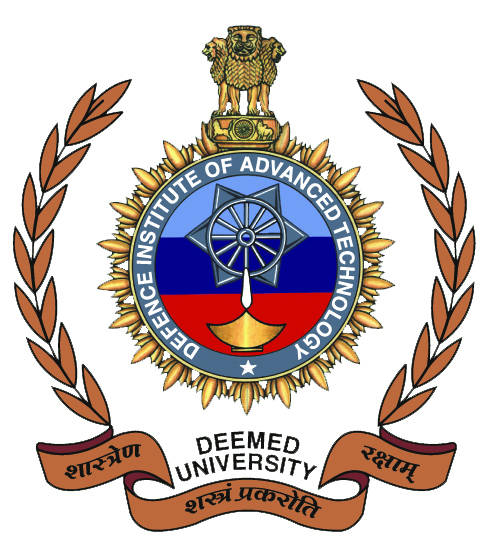
Deemed University
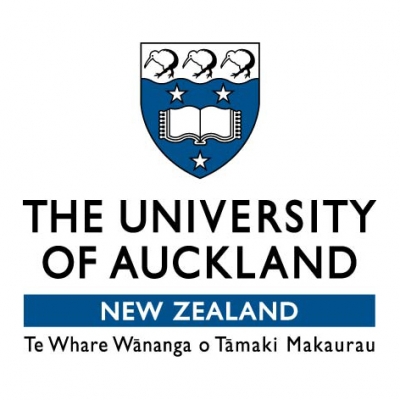
Auckland University
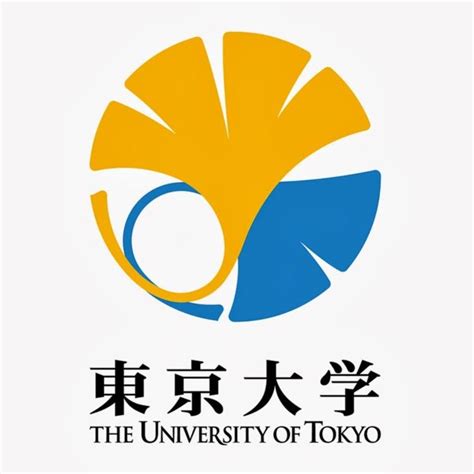
The University of Tokyo
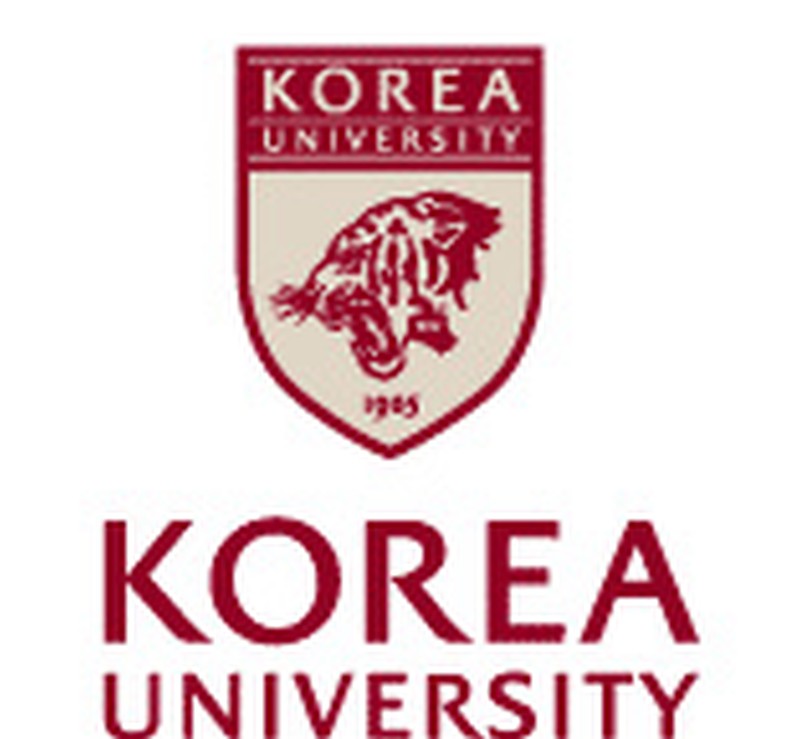
Korea University
Ki: 1.6 nM
PKA inhibitor fragment (6-22) amide is a PKA inhibitor.
In cell biology, protein kinase A (PKA) is a family of enzymes whose activity is dependent on cellular levels of cyclic AMP (cAMP). PKA is also regarded as a cAMP-dependent protein kinase. PKA has various functions in the cell, such as regulation of sugar, glycogen, and lipid metabolism.
In vitro: Compared with (Ala)Kemptide, PKA inhibitor fragment (6-22) amide was found to have significant difference in inhibitory potency, likely due to the critical role of several of the nonarginine residues to this difference in potency. The minimal length analog of PKA inhibitor fragment (6-22) that inhibited CAMP-dependent protein kinase with high potency was the 17-residue PKI-(6-22)-amide. PKA inhibitor fragment (6-22) interacted at the peptide/protein binding portion of the active site in a competitive manner with a low nanomolar Ki value. To inhibit CAMP-dependent protein kinase, PKA inhibitor fragment (6-22) required both the pseudosubstrate site (residues 14-22) and additional NH2-terminal determinants within residues 6-13. PKA inhibitor fragment (6-22) could clear mimic protein and peptide substrates in interacting with the binding region of the active site particularly in the COOH-terminal pseudosubstrate basic domain. Undoubtedly, the overall affinity of PKA inhibitor fragment (6-22) was due to the number of hydrogen bonds as well as other bonding interactions with the active site of the CAMP-dependent protein kinase [1, 2].
In vivo: N/A
Clinical trial: N/A
References:
[1] Glass et al (1989) Primary structural determinants essential for potent inhibition of cAMP-dependent protein kinase by inhibitory peptides corresponding to the active portion of the heat-stable inhibitor protein. J.Biol.Chem. 264 8802. PMID: 2722799.
[2] Glass et al (1989) Protein kinase inhibitor-(6-22)-amide peptide analogs with standard and nonstandard amino acid substitutions for phenylalanine 10. J.Biol.Chem. 264 14579. PMID: 2760075.
- Dihydrodaidzin
Catalog No.:BCN2879
CAS No.:121927-96-6
- Sophoraflavanone C
Catalog No.:BCN3543
CAS No.:121927-91-1
- Aurothioglucose
Catalog No.:BCC5446
CAS No.:12192-57-3
- CCT 031374 hydrobromide
Catalog No.:BCC6258
CAS No.:1219184-91-4
- (-)-MK 801
Catalog No.:BCC4593
CAS No.:121917-57-5
- Dorsomorphin 2HCl
Catalog No.:BCC4361
CAS No.:1219168-18-9
- GKT137831
Catalog No.:BCC5460
CAS No.:1218942-37-0
- LDE225 Diphosphate
Catalog No.:BCC1693
CAS No.:1218778-77-8
- CAY10505
Catalog No.:BCC4990
CAS No.:1218777-13-9
- Pidotimod
Catalog No.:BCC4823
CAS No.:121808-62-6
- Xylometazoline HCl
Catalog No.:BCC4879
CAS No.:1218-35-5
- Ac-Trp-OH
Catalog No.:BCC3109
CAS No.:1218-34-4
- 4,5-Diepipsidial A
Catalog No.:BCN3920
CAS No.:1219603-97-0
- PF 4778574
Catalog No.:BCC6322
CAS No.:1219633-99-4
- (±)-Anatoxin A fumarate
Catalog No.:BCC6796
CAS No.:1219922-30-1
- Sulfadimethoxine
Catalog No.:BCC5159
CAS No.:122-11-2
- Tetraethoxypropane
Catalog No.:BCN2221
CAS No.:122-31-6
- Glycerine trioleate
Catalog No.:BCN2287
CAS No.:122-32-7
- Zingerone
Catalog No.:BCN1192
CAS No.:122-48-5
- (-)-Ampelopsin H
Catalog No.:BCC8842
CAS No.:
- Cinnamyl cinnamate
Catalog No.:BCN4722
CAS No.:122-69-0
- Phenylacetaldehyde
Catalog No.:BCN3819
CAS No.:122-78-1
- 3-Phenyl-1-propanol
Catalog No.:BCC8102
CAS No.:122-97-4
- Sulfamonomethoxine
Catalog No.:BCC9156
CAS No.:1220-83-3
Cav1.2 channel current block by the PKA inhibitor H-89 in rat tail artery myocytes via a PKA-independent mechanism: Electrophysiological, functional, and molecular docking studies.[Pubmed:28583845]
Biochem Pharmacol. 2017 Sep 15;140:53-63.
To characterize the role of cAMP-dependent protein kinase (PKA) in regulating vascular Ca(2+) current through Cav1.2 channels [ICa1.2], we have documented a marked capacity of the isoquinoline H-89, widely used as a PKA inhibitor, to reduce current amplitude. We hypothesized that the ICa1.2 inhibitory activity of H-89 was mediated by mechanisms unrelated to PKA inhibition. To support this, an in-depth analysis of H-89 vascular effects on both ICa1.2 and contractility was undertaken by performing whole-cell patch-clamp recordings and functional experiments in rat tail main artery single myocytes and rings, respectively. H-89 inhibited ICa1.2 with a pIC50 (M) value of about 5.5, even under conditions where PKA activity was either abolished by both the PKA antagonists KT5720 and protein kinase inhibitor fragment 6-22 amide or enhanced by the PKA stimulators 6-Bnz-cAMP and 8-Br-cAMP. Inhibition of ICa1.2 by H-89 appeared almost irreversible upon washout, was charge carrier- and voltage-dependent, and antagonised by the Cav1.2 channel agonist (S)-(-)-Bay K 8644. H-89 did not alter both potency and efficacy of verapamil, did not affect current kinetics or voltage-dependent activation, while shifting to the left the 50% voltage of inactivation in a concentration-dependent manner. H-89 docked at the alpha1C subunit in a pocket region close to that of (S)-(-)-Bay K 8644 docking, forming a hydrogen bond with the same, key amino acid residue Tyr-1489. Finally, both high K(+)- and (S)-(-)-Bay K 8644-induced contractions of rings were fully reverted by H-89. In conclusion, these results indicate that H-89 inhibited vascular ICa1.2 and, consequently, the contractile function through a PKA-independent mechanism. Therefore, caution is recommended when interpreting experiments where H-89 is used to inhibit vascular smooth muscle PKA.
Adenosine modulates hypoxia-induced responses in rat PC12 cells via the A2A receptor.[Pubmed:9490823]
J Physiol. 1998 Apr 1;508 ( Pt 1):95-107.
1. The present study was undertaken to determine the role of adenosine in mediating the cellular responses to hypoxia in rat phaeochromocytoma (PC12) cells, an oxygen-sensitive clonal cell line. 2. Reverse transcriptase polymerase chain reaction studies revealed that PC12 cells express adenosine deaminase (the first catalysing enzyme of adenosine degradation) and the A2A and A2B adenosine receptors, but not the A1 or A3 adenosine receptors. 3. Whole-cell current- and voltage-clamp experiments showed that adenosine attenuated the hypoxia-induced membrane depolarization. The hypoxia-induced suppression of the voltage-sensitive potassium current (IK(V)) was markedly reduced by adenosine. Furthermore, extracellularly applied adenosine increased the peak amplitudes of IK(V) in a concentration-dependent manner. This increase was blocked by pretreatment not only with a non-specific adenosine receptor antagonist, 8-phenyltheophylline (8-PT), but also with a selective A2A receptor antagonist, ZM241385. 4. Ca2+ imaging studies using fura-2 acetoxymethyl ester (fura-2 AM) revealed that the increase in intracellular free Ca2+ during hypoxic exposure was attenuated significantly by adenosine. Voltage-clamp studies showed that adenosine inhibited the voltage-dependent Ca2+ currents (ICa) in a concentration-dependent fashion. This inhibition was also abolished by both 8-PT and ZM241385. 5. The modulation of both IK(V) and ICa by adenosine was prevented by intracellular application of an inhibitor of protein kinase A (PKA), PKA inhibitor fragment (6-22) amide. In addition, the effect of adenosine on either IK(V) or ICa was absent in PKA-deficient PC12 cells. 6. These results indicate that the modulatory effects of adenosine on the hypoxia-induced membrane responses of PC12 cells are likely to be mediated via activation of the A2A receptor, and that the PKA pathway is required for these modulatory actions. We propose that this modulation serves to regulate membrane excitability in PC12 cells and possibly other oxygen-sensitive cells during hypoxia.
Differential modulation of evoked and spontaneous glycine release from rat spinal cord glycinergic terminals by the cyclic AMP/protein kinase A transduction cascade.[Pubmed:15485496]
J Neurochem. 2004 Nov;91(3):657-66.
The mechanisms underlying cyclic AMP modulation of action potential-dependent and -independent (spontaneous) release of glycine from terminals synapsing onto sacral dorsal commissural nucleus neurons of lamina X were studied in spinal cord slices using conventional patch-clamp recordings. 3-Isobutyl-1-methylxanthine (IBMX), a phosphodiesterase inhibitor, and forskolin increased the amplitude of evoked inhibitory postsynaptic currents (eIPSCs) in a sensitive manner to protein kinase A (PKA) inhibition (with KT-5720). Direct activation (with adenosine 3',5'-cyclic-monophosphothioate, Sp-isomer) and inhibition (with adenosine 3',5'-cyclic-monophosphothioate, Rp-isomer) of PKA increased and decreased the eIPSC amplitude, respectively. Paired pulse experiments and direct injection of PKA inhibitor fragment 6-22 amide (PKI(6-22)) into the recording neuron revealed that these effects on eIPSC amplitude occurred presynaptically, indicating that evoked glycine release is regulated by presynaptic cAMP via changes in PKA activity. Increasing cAMP also increased spontaneous release of glycine, causing an increased frequency of miniature IPSCs (mIPSCs). In contrast to the effects on evoked release, this response was not solely mediated via PKA, as it was not occluded by PKA inhibition, and both direct inhibition and direct activation of PKA actually enhanced mIPSC frequency. Direct inhibition of cAMP (with SQ 22536) did, however, reduce mIPSC frequency. These results suggest cAMP modulation of evoked and spontaneous release involves different presynaptic mechanisms and proteins.
Prostaglandin E1 activates the large-conductance KCa channel in human corporal smooth muscle cells.[Pubmed:10467518]
Int J Impot Res. 1999 Aug;11(4):189-99.
The large conductance calcium-sensitive potassium channel (KCa or maxi-K) is an important modulator of human corporal smooth muscle tone, and therefore, erectile capacity. The goal of this investigation was to evaluate the actions of prostaglandin E1 (PGE1), the most widely used and effective drug for the treatment of impotence, on the activity of the KCa channel, a prominent K+ current present in human corporal smooth muscle. Whole-cell patch clamp studies conducted on short-term cultured and enzymatically dissociated human corporal smooth muscle cells, revealed mean resting potentials of -50.8 +/- 2.1 mV (n = 8) and -34 +/- 4 mV (n = 8), respectively. In the attached-patch configuration, the corresponding single-channel slope conductance values for the KCa channel subtype were 173 +/- 4 pS (n = 8) in cultured cells, and 190 +/- 13 pS (n = 3) in freshly isolated myocytes. Furthermore, voltage clamp experiments revealed that relative to control values, the application of PGE1 to cultured cells (3.3 or 33 microM) elicited an apparent increase in both the open probability (Po; ranging from 1.2-23 fold), and the mean open time (5-6 fold) of the KCa channel at membrane potentials of +90 mV and +110 mV. PGE1-induced alterations in KCa channel activity were also observed in freshly isolated corporal myocytes. In the whole cell-recording mode, statistically significant, Charybdotoxin-sensitive (100 nM) 2-3 fold increases in the outward K+ currents were observed in both cultured and freshly isolated corporal myocytes. The presence of a PKA inhibitor (fragment 6-22 amide; 10 microM) in the pipette tip was also associated with a nearly complete ablation of the observed PGE1-induced whole cell K+ currents. Taken together, these data confirm and extend our previous observations, and indicate that PGE1-induced relaxation of human corporal smooth muscle is related, at least in part, to activation of the KCa channel subtype resulting in cellular hyperpolarization.
Inhibition of the cAMP pathway decreases early long-term potentiation at CA1 hippocampal synapses.[Pubmed:10844013]
J Neurosci. 2000 Jun 15;20(12):4446-51.
Long-term potentiation (LTP) has several different phases, and there is general agreement that the late phase of LTP requires the activation of adenylyl cyclase (AC) and cAMP-dependent protein kinase (PKA). In contrast, several studies indicate that the early LTP is not affected by interfering with the cAMP pathway. We have further tested the role of the cAMP pathway in early LTP using several types of inhibitors. Bath application of the PKA inhibitor H89 suppressed the early LTP induced by a single tetanus. Similarly, the LTP induced by a pairing protocol was decreased by postsynaptic intracellular perfusion of the peptide PKA inhibitor PKI(6-22) amide. The decrease of LTP produced by these inhibitors was evident immediately after induction. These results indicate that PKA is important in early LTP, that its locus of action is postsynaptic, and that it does not act merely by enhancing the depolarization required for LTP induction. The failure of some other inhibitors of the cAMP pathway to affect the early phase of LTP might be attributable to the saturation of some step in the cAMP pathway during a tetanus. In agreement with this hypothesis we found that application of the AC inhibitor SQ 22536 by itself did not affect the early phase of LTP, but did produce a reduction if the cAMP pathway was already attenuated by the PKA inhibitor H89. Our analysis of the results of genetic modifications of the cAMP pathway, especially the work on AC knock-outs, indicates that the genetic data are generally consistent with the pharmacological results showing the importance of this pathway in early LTP.
Primary structural determinants essential for potent inhibition of cAMP-dependent protein kinase by inhibitory peptides corresponding to the active portion of the heat-stable inhibitor protein.[Pubmed:2722799]
J Biol Chem. 1989 May 25;264(15):8802-10.
PKI-(5-24)-amide is a 20-residue peptide with the sequence, Thr5-Thr-Tyr-Ala-Asp-Phe-Ile-Ala-Ser-Gly-Arg-Thr-Gly-Arg-Arg-Asn-A la-Ile-His- Asp24-NH2, that corresponds to the active portion of the heat-stable inhibitor protein of cAMP-dependent protein kinase (Cheng, H.-C., Kemp, B. E., Pearson, R. B., Smith, A. J., Misconi, L., Van Patten, S. M., and Walsh, D. A. (1986) J. Biol. Chem. 261, 989-992). Amino acid residues in PKI-(5-24)-amide responsible for the potent inhibition (Ki = 2.3 nM) of the catalytic subunit of protein kinase were further investigated using deletion and substitution analogs of the synthetic peptide. Residues 5, 23, and 24 were not required for activity since the 17-residue PKI-(6-22)-amide retained full potency. Sequential removal of the first seven amino acids from the NH2 terminus of PKI-(5-24)-amide caused a progressive 50-fold loss of inhibitory potency. In contrast, substitution of either Thr6, Asp9, or Ile11 with alanine, or Ala8 by leucine, in PKI-(5-22)-amide produced less than 3-fold decreases in potency. Of the 2 aromatic residues in PKI-(5-22)-amide, the individual substitution of Phe10 and Tyr7 by alanine caused, respectively, 90- and 5-fold decreases in inhibitory potency, demonstrating important roles for each. This NH2-terminal portion of the peptide is believed to contain a significant portion of alpha-helix. Many recognition or structural determinants are also essential in the COOH-terminal portion of PKI-(5-22)-amide. In addition to the basic subsite provided by the three arginines, several other of the residues are critical for full inhibitory potency. Substitution of Ile22 by glycine in either PKI-(5-22)-amide or PKI-(14-22)-amide lowered the inhibitory potency by 150- and 50-fold, respectively. Separate replacement of Gly17 or Asn20, in either PKI-(5-22)-amide or PKI-(14-22)-amide, caused 7-15-fold decreases in potency. Substitution of both Gly17 and Asn20 together (in PKI-(14-22)-amide) produced a synergistic loss of inhibitory activity. [Leu13,Ile14]PKI-(5-22)-amide, a doubly substituted analog exhibited a 42-fold increase in Ki value. We conclude that Ser13 and/or Gly14, Gly17, Asn20, and Ile22 each contribute important features to the binding of these inhibitory peptides to the protein kinase, either by providing recognition determinants, inducing structure, and/or allowing essential peptide backbone flexibility.(ABSTRACT TRUNCATED AT 400 WORDS)
Protein kinase inhibitor-(6-22)-amide peptide analogs with standard and nonstandard amino acid substitutions for phenylalanine 10. Inhibition of cAMP-dependent protein kinase.[Pubmed:2760075]
J Biol Chem. 1989 Aug 25;264(24):14579-84.
The minimal structure in the heat-stable inhibitor protein of cAMP-dependent protein kinase required for a low nanomolar potency of inhibition is the peptide Thr6-Tyr-Ala-Asp-Phe-Ile-Ala-Ser-Gly-Arg-Thr-Gly-Arg-Arg-Asn-Ala-+ ++Ile22-NH2 (PKI-(6-22)-amide). While primary structural determinants for interaction with the protein kinase are distributed throughout the 17 residues of this peptide, we have previously shown that phenylalanine 10 in the NH2-terminal portion is a particularly important determinant for high affinity binding (Glass, D. B., Cheng, H.-C., Mende-Mueller, L., Reed, J., and Walsh, D. A. (1989) J. Biol. Chem. 264, 8802-8810). To investigate this requirement further, peptide analogs of PKI-(6-22)-amide in which various natural and nonstandard amino acids are substituted for phenylalanine 10 have been synthesized and tested for inhibitory potency against the catalytic subunit of the protein kinase. Consistent with the importance of the hydrophobicity of phenylalanine, an alanine 10 substitution analog exhibited a 270-fold decrease in inhibitory potency, whereas the leucine 10 analog lost only 33-fold in activity as compared to the parent peptide PKI-(6-22)-amide. Peptides containing the spatial conformation analogs D-phenylalanine, homophenylalanine, or phenylglycine were 60-120-fold less potent than the parent peptide. Peptides containing various para-substituted phenylalanines at position 10 were only 5-11-fold less potent. One exception to this was (4'-azidophenylalanine 10)PKI-(6-22)-amide, which was nearly equipotent with the parent inhibitor. The most potent analogs were those peptides containing highly aromatic residues at position 10. The 2'-thienylalanine 10, tryptophan (formyl) 10, tryptophan 10, and the 1'-naphthylalanine 10 analogs were 3-fold less potent, equipotent, slightly more potent, and 4-fold more potent than the parent peptide inhibitor, respectively. We conclude that phenylalanine 10 in PKI-(6-22)-amide, and presumably in the native protein inhibitor, interacts through specific hydrophobic and/or aromatic binding to a hydrophobic pocket or cleft near the active site of the protein kinase.