3-QuinuclidinoneCAS# 3731-38-2 |
Quality Control & MSDS
Number of papers citing our products
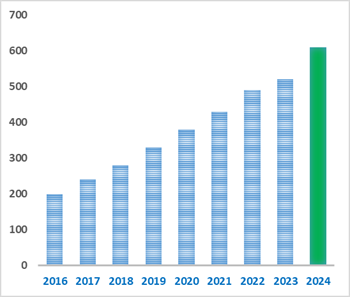
Chemical structure

3D structure
Cas No. | 3731-38-2 | SDF | Download SDF |
PubChem ID | 19507 | Appearance | Powder |
Formula | C7H11NO | M.Wt | 125.2 |
Type of Compound | N/A | Storage | Desiccate at -20°C |
Solubility | Soluble in Chloroform,Dichloromethane,Ethyl Acetate,DMSO,Acetone,etc. | ||
Chemical Name | 1-azabicyclo[2.2.2]octan-3-one | ||
SMILES | C1CN2CCC1C(=O)C2 | ||
Standard InChIKey | ZKMZPXWMMSBLNO-UHFFFAOYSA-N | ||
Standard InChI | InChI=1S/C7H11NO/c9-7-5-8-3-1-6(7)2-4-8/h6H,1-5H2 | ||
General tips | For obtaining a higher solubility , please warm the tube at 37 ℃ and shake it in the ultrasonic bath for a while.Stock solution can be stored below -20℃ for several months. We recommend that you prepare and use the solution on the same day. However, if the test schedule requires, the stock solutions can be prepared in advance, and the stock solution must be sealed and stored below -20℃. In general, the stock solution can be kept for several months. Before use, we recommend that you leave the vial at room temperature for at least an hour before opening it. |
||
About Packaging | 1. The packaging of the product may be reversed during transportation, cause the high purity compounds to adhere to the neck or cap of the vial.Take the vail out of its packaging and shake gently until the compounds fall to the bottom of the vial. 2. For liquid products, please centrifuge at 500xg to gather the liquid to the bottom of the vial. 3. Try to avoid loss or contamination during the experiment. |
||
Shipping Condition | Packaging according to customer requirements(5mg, 10mg, 20mg and more). Ship via FedEx, DHL, UPS, EMS or other couriers with RT, or blue ice upon request. |

3-Quinuclidinone Dilution Calculator

3-Quinuclidinone Molarity Calculator
1 mg | 5 mg | 10 mg | 20 mg | 25 mg | |
1 mM | 7.9872 mL | 39.9361 mL | 79.8722 mL | 159.7444 mL | 199.6805 mL |
5 mM | 1.5974 mL | 7.9872 mL | 15.9744 mL | 31.9489 mL | 39.9361 mL |
10 mM | 0.7987 mL | 3.9936 mL | 7.9872 mL | 15.9744 mL | 19.9681 mL |
50 mM | 0.1597 mL | 0.7987 mL | 1.5974 mL | 3.1949 mL | 3.9936 mL |
100 mM | 0.0799 mL | 0.3994 mL | 0.7987 mL | 1.5974 mL | 1.9968 mL |
* Note: If you are in the process of experiment, it's necessary to make the dilution ratios of the samples. The dilution data above is only for reference. Normally, it's can get a better solubility within lower of Concentrations. |
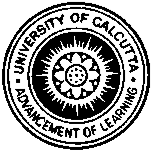
Calcutta University
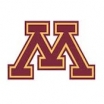
University of Minnesota
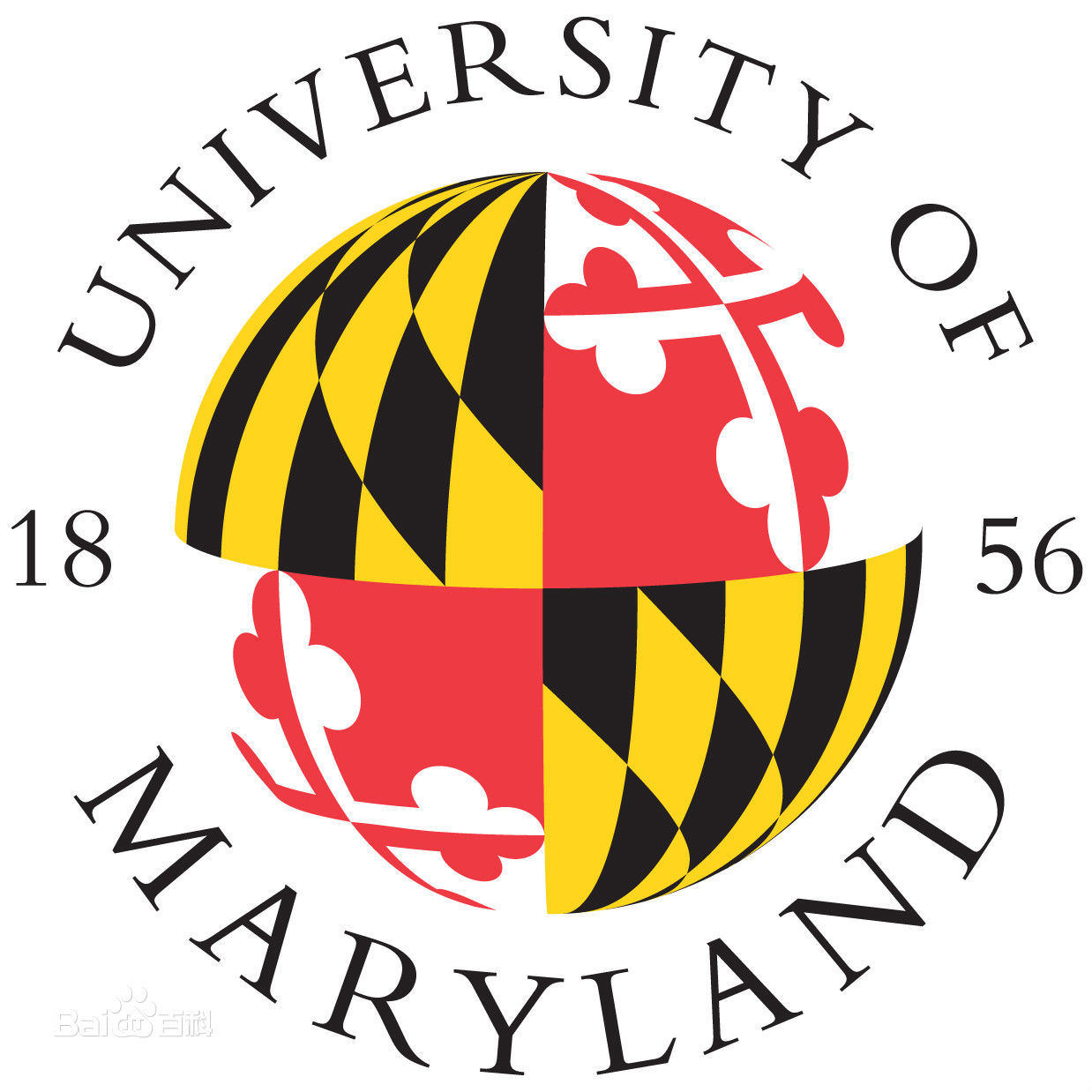
University of Maryland School of Medicine
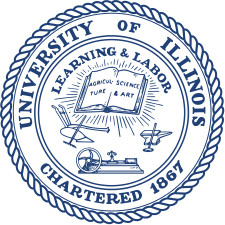
University of Illinois at Chicago
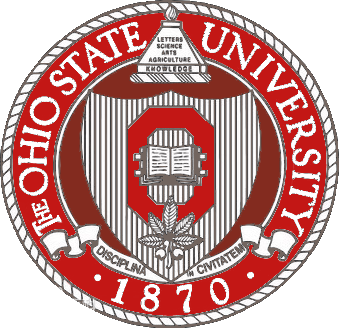
The Ohio State University
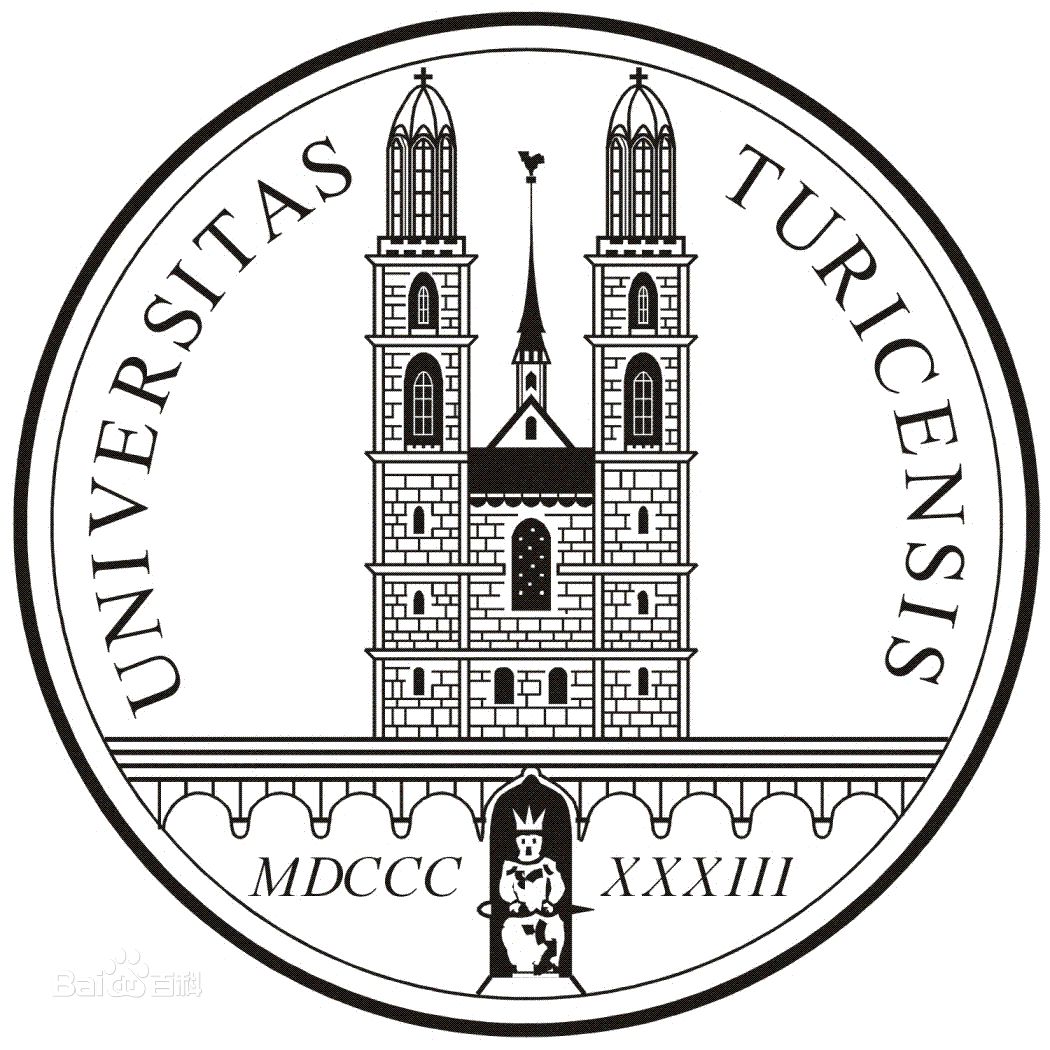
University of Zurich
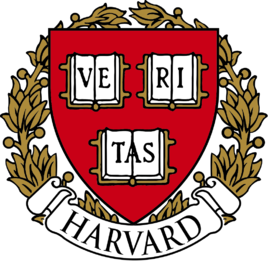
Harvard University
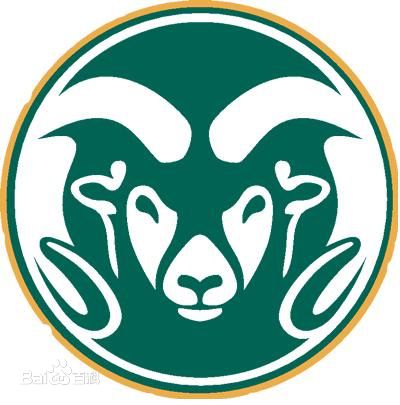
Colorado State University
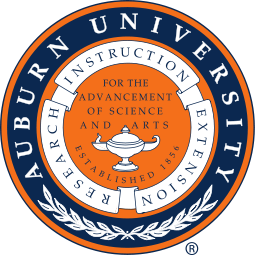
Auburn University
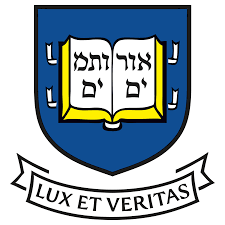
Yale University
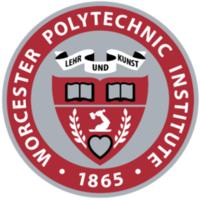
Worcester Polytechnic Institute
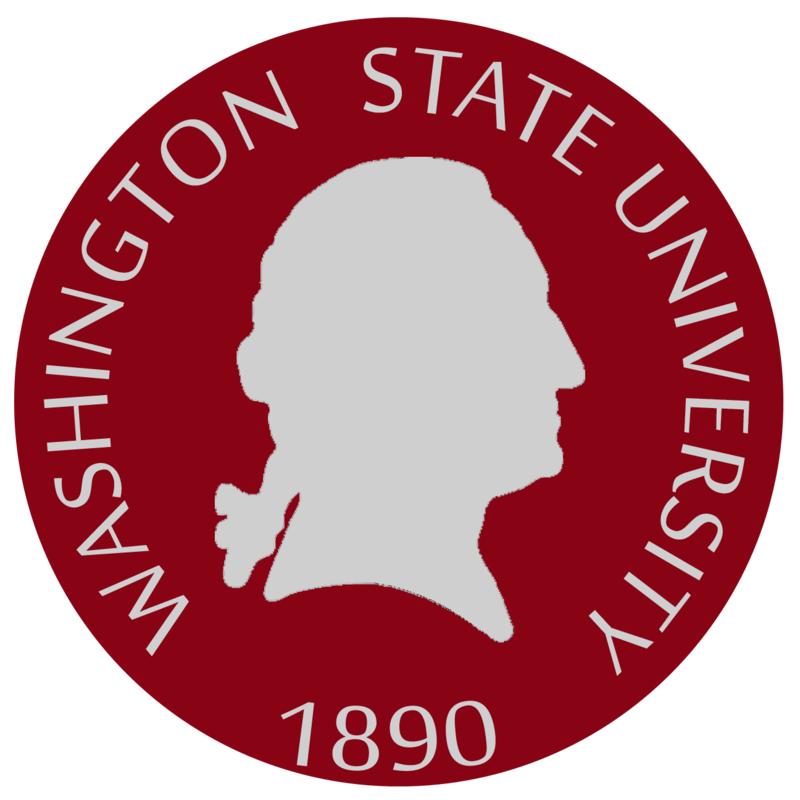
Washington State University
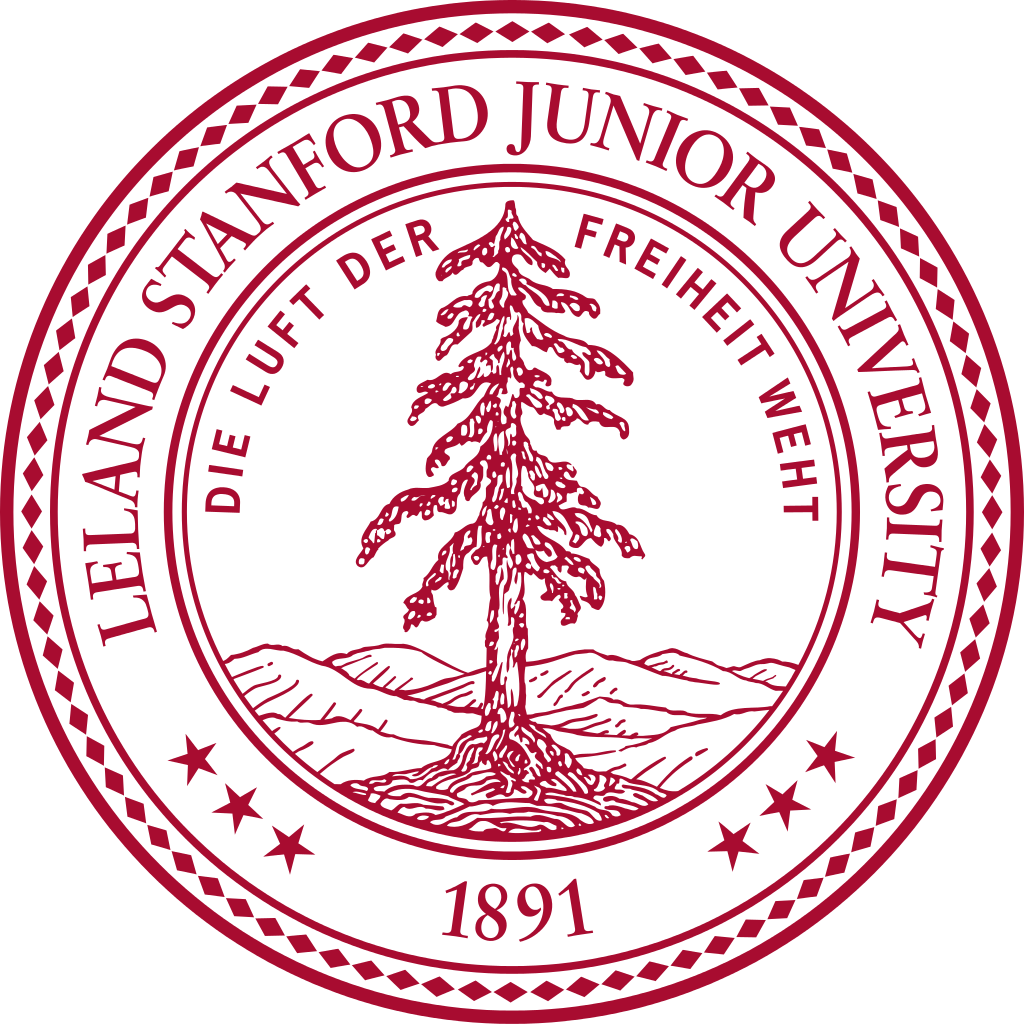
Stanford University
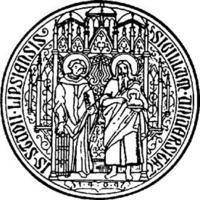
University of Leipzig
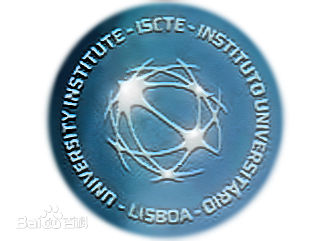
Universidade da Beira Interior
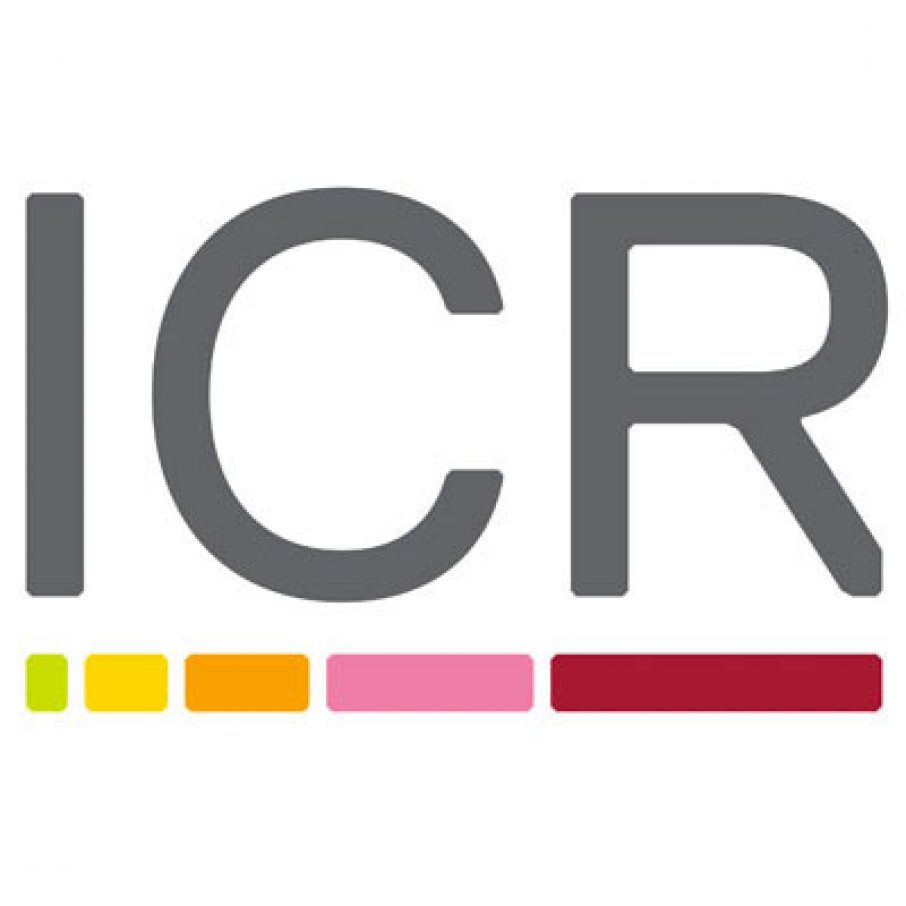
The Institute of Cancer Research
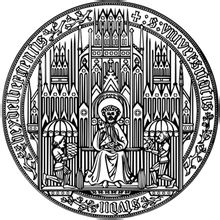
Heidelberg University
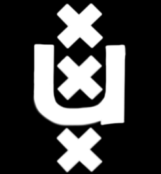
University of Amsterdam
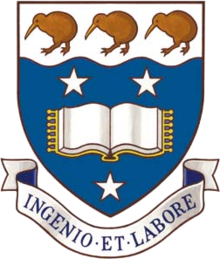
University of Auckland
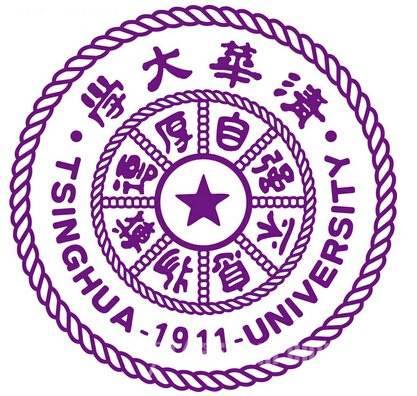
TsingHua University
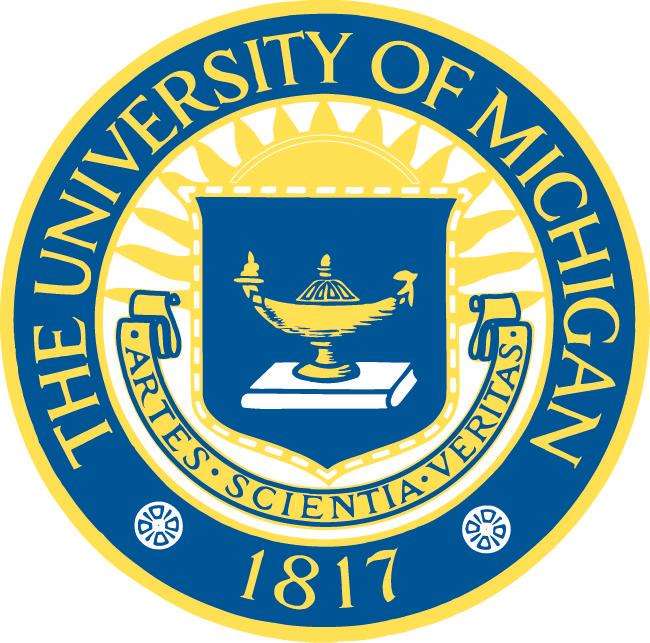
The University of Michigan
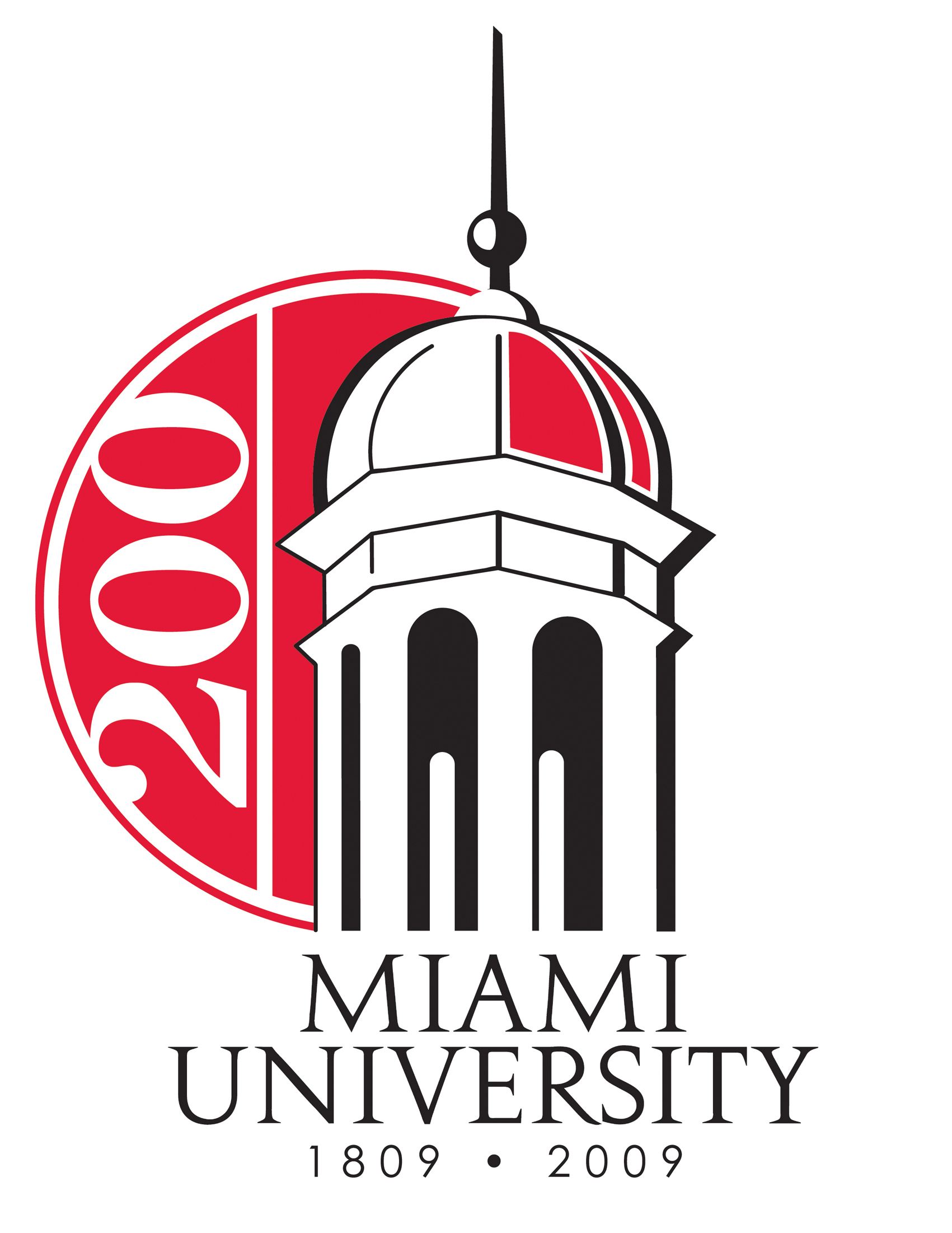
Miami University
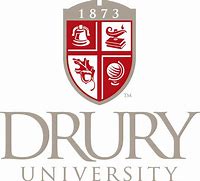
DRURY University
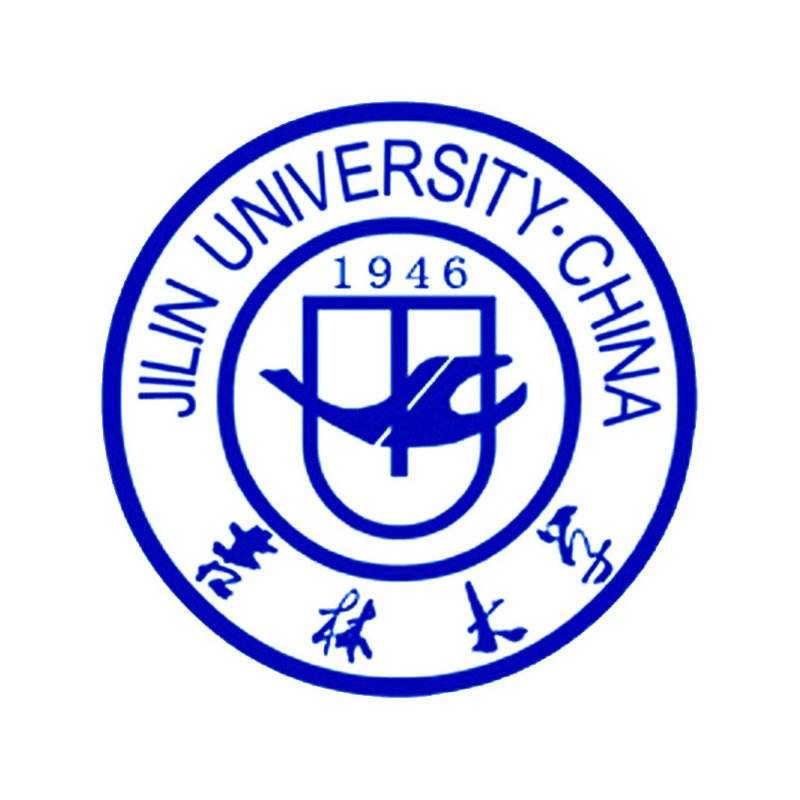
Jilin University
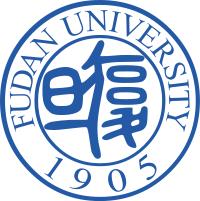
Fudan University
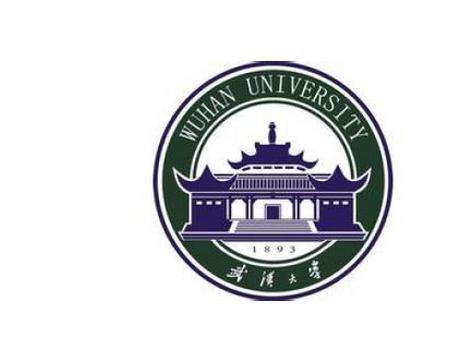
Wuhan University
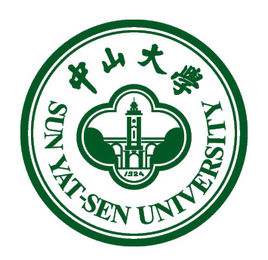
Sun Yat-sen University
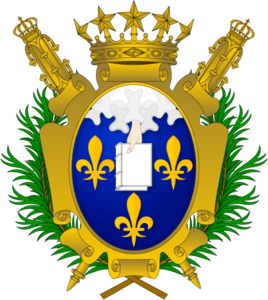
Universite de Paris
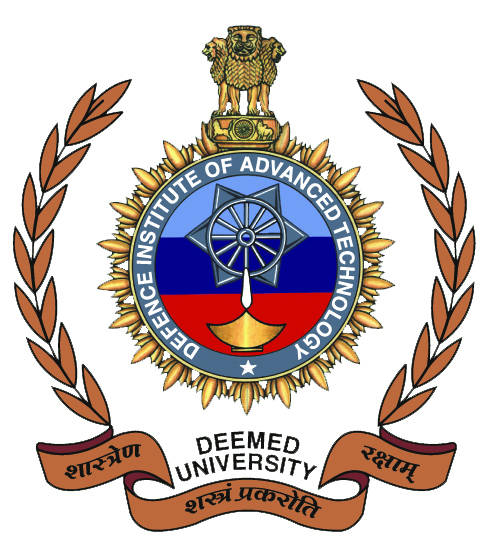
Deemed University
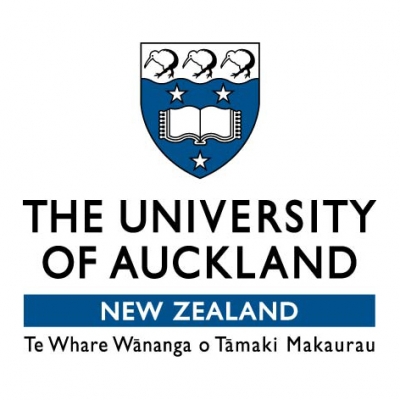
Auckland University
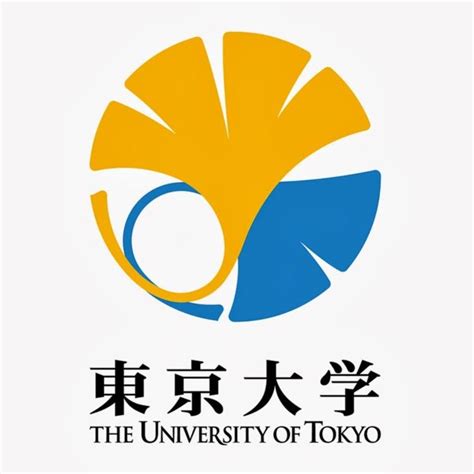
The University of Tokyo
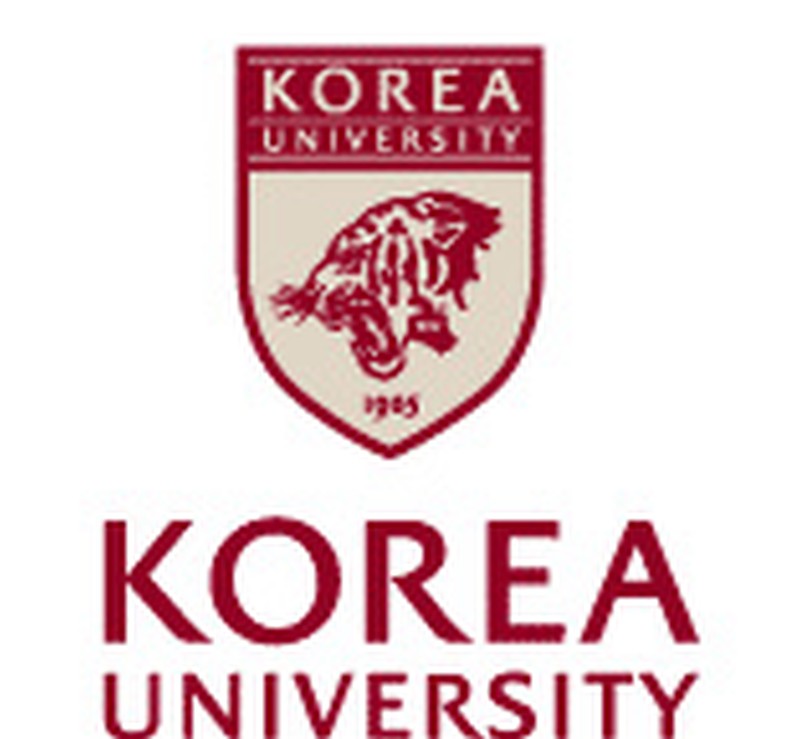
Korea University
- Flavokawain C
Catalog No.:BCN8456
CAS No.:37308-75-1
- H-D-Tyr-OMe.HCl
Catalog No.:BCC3135
CAS No.:3728-20-9
- Sennoside D
Catalog No.:BCN1005
CAS No.:37271-17-3
- Sennoside C
Catalog No.:BCN1004
CAS No.:37271-16-2
- TCS OX2 29
Catalog No.:BCC7670
CAS No.:372523-75-6
- Wilfordine
Catalog No.:BCN3083
CAS No.:37239-51-3
- Wilfortrine
Catalog No.:BCN3085
CAS No.:37239-48-8
- Wilforgine
Catalog No.:BCN5427
CAS No.:37239-47-7
- Sieber Linker
Catalog No.:BCC2835
CAS No.:3722-51-8
- PIK-75
Catalog No.:BCC1163
CAS No.:372196-77-5
- Citromycin
Catalog No.:BCN7459
CAS No.:37209-30-6
- Capsidiol
Catalog No.:BCC8140
CAS No.:37208-05-2
- Decloxizine
Catalog No.:BCC5529
CAS No.:3733-63-9
- Cephaeline Hydrochloride
Catalog No.:BCC8307
CAS No.:3738-70-3
- DS2
Catalog No.:BCC7748
CAS No.:374084-31-8
- Boc-DL-Ala-OH
Catalog No.:BCC3050
CAS No.:3744-87-4
- Istaroxime hydrochloride
Catalog No.:BCC1661
CAS No.:374559-48-5
- Kisspeptin 10 (human)
Catalog No.:BCC7415
CAS No.:374675-21-5
- Metastin (human)
Catalog No.:BCC5761
CAS No.:374683-24-6
- DHP Linker
Catalog No.:BCC2830
CAS No.:3749-36-8
- Amikacin
Catalog No.:BCC5206
CAS No.:37517-28-5
- 2-Chlorocinnamic acid
Catalog No.:BCN5036
CAS No.:3752-25-8
- LY451395
Catalog No.:BCC5377
CAS No.:375345-95-2
- H-D-Ala(4-pyridyl)-OH.HCl
Catalog No.:BCC3325
CAS No.:37535-49-2
p53 reactivation with induction of massive apoptosis-1 (PRIMA-1) inhibits amyloid aggregation of mutant p53 in cancer cells.[Pubmed:30602570]
J Biol Chem. 2019 Mar 8;294(10):3670-3682.
p53 mutants can form amyloid-like structures that accumulate in cells. p53 reactivation with induction of massive apoptosis-1 (PRIMA-1) and its primary active metabolite, 2-methylene-3-Quinuclidinone (MQ), can restore unfolded p53 mutants to a native conformation that induces apoptosis and activates several p53 target genes. However, whether PRIMA-1 can clear p53 aggregates is unclear. In this study, we investigated whether PRIMA-1 can restore aggregated mutant p53 to a native form. We observed that the p53 mutant protein is more sensitive to both PRIMA-1 and MQ aggregation inhibition than WT p53. The results of anti-amyloid oligomer antibody assays revealed that PRIMA-1 reverses mutant p53 aggregate accumulation in cancer cells. Size-exclusion chromatography of the lysates from mutant p53-containing breast cancer and ovarian cell lines confirmed that PRIMA-1 substantially decreases p53 aggregates. We also show that MDA-MB-231 cell lysates can "seed" aggregation of the central core domain of recombinant WT p53, corroborating the prion-like behavior of mutant p53. We also noted that this aggregation effect was inhibited by MQ and PRIMA-1. This study provides the first demonstration that PRIMA-1 can rescue amyloid-state p53 mutants, a strategy that could be further explored as a cancer treatment.
Facile fabrication of 3D porous hybrid sphere by co-immobilization of multi-enzyme directly from cell lysates as an efficient and recyclable biocatalyst for asymmetric reduction with coenzyme regeneration in situ.[Pubmed:28526345]
Int J Biol Macromol. 2017 Oct;103:424-434.
Ni(2+)-agarose bead-wrapped multi-enzyme/inorganic hybrid sphere composed of the immobilized enzymes as organic component and NaH2PO4 and NaCl as inorganic component was developed by co-immobilizing extracellular His-tagged 3-Quinuclidinone reductases and glucose dehydrogenase without pre-purification. The resulting biocatalysts has 3D porous architectures as confirmed by SEM and FESEM, and it enabled the continuous biotransformation of 3-quinuclidone to (R)-3-quinuclidinol with cofactor regeneration in situ. The 3D porous biocatalysts were formed via three steps: First, immobilization of the His-tagged enzymes directly from the cell lysates supernatant. Next, formation of enzyme aggregates, ribbons and gels. Finally, the enzymes, the formed aggregates/ribbons/gels and salt were incorporated to the foam and then covered the Ni(2+)-agarose bead. The technique made the immobilization of these enzymes effective such that specific enzyme loading of 60.8mg/g support and enzyme loading efficiency of 92.3% were achieved. As a direct consequence, the biocatalyst catalyzed the conversion of 3-Quinuclidinone (204g/L) to (R)-3-quinuclidinol in 100% yield and 100% ee at 4.5h, and the recyclability of the biocatalyst was excellent, retaining>95% conversion yield and 100% ee even after the fifteenth runs. Overall, our strategy is demonstrated to be a promising method for developing efficient and robust biocatalyst for asymmetric synthesis.
Functional characterisation of a tropine-forming reductase gene from Brugmansia arborea, a woody plant species producing tropane alkaloids.[Pubmed:26988730]
Phytochemistry. 2016 Jul;127:12-22.
Brugmansia arborea is a woody plant species that produces tropane alkaloids (TAs). The gene encoding tropine-forming reductase or tropinone reductase I (BaTRI) in this plant species was functionally characterised. The full-length cDNA of BaTRI encoded a 272-amino-acid polypeptide that was highly similar to tropinone reductase I from TAs-producing herbal plant species. The purified 29kDa recombinant BaTRI exhibited maximum reduction activity at pH 6.8-8.0 when tropinone was used as substrate; it also exhibited maximum oxidation activity at pH 9.6 when tropine was used as substrate. The Km, Vmax and Kcat values of BaTRI for tropinone were 2.65mM, 88.3nkatmg(-1) and 2.93S(-1), respectively, at pH 6.4; the Km, Vmax and Kcat values of TRI from Datura stramonium (DsTRI) for tropinone were respectively 4.18mM, 81.20nkatmg(-1) and 2.40S(-1) at pH 6.4. At pH 6.4, 6.8 and 7.0, BaTRI had a significantly higher activity than DsTRI. Analogues of tropinone, 4-methylcyclohexanone and 3-Quinuclidinone hydrochloride, were also used to investigate the enzymatic kinetics of BaTRI. The Km, Vmax and Kcat values of BaTRI for tropine were 0.56mM, 171.62nkat.mg(-1) and 5.69S(-1), respectively, at pH 9.6; the Km, Vmax and Kcat values of DsTRI for tropine were 0.34mM, 111.90nkatmg(-1) and 3.30S(-1), respectively, at pH 9.6. The tissue profiles of BaTRI differed from those in TAs-producing herbal plant species. BaTRI was expressed in all examined organs but was most abundant in secondary roots. Finally, tropane alkaloids, including hyoscyamine, anisodamine and scopolamine, were detected in various organs of B. arborea by HPLC. Interestingly, scopolamine constituted most of the tropane alkaloids content in B. arborea, which suggests that B. arborea is a scopolamine-rich plant species. The scopolamine content was much higher in the leaves and stems than in other organs. The gene expression and TAs accumulation suggest that the biosynthesis of hyoscyamine, especially scopolamine, occurred not only in the roots but also in the aerial parts of B. arborea.
Ruthenium and osmium complexes of hemilabile chiral monophosphinite ligands derived from 1D-pinitol or 1D-chiro-inositol as catalysts for asymmetric hydrogenation reactions.[Pubmed:25315464]
Dalton Trans. 2014 Dec 7;43(45):17163-71.
The monophosphinite ligands, 1D-1,2;5,6-di-O-cyclopentylidene-3-O-methyl-4-O-diphenylphosphino-chiro-inositol (D-P1), 1D-1,2;5,6-di-O-isopropylidene-3-O-methyl-4-O-diphenylphosphino-chiro-inositol (D-P2), 1D-1,2;5,6-di-O-cyclohexylidene-3-O-methyl-4-O-diphenylphosphino-chiro-inositol (D-P3), and 1D-1,2;5,6-di-O-cyclopentylidene-3-O-ethyl-4-O-diphenylphosphino-chiro-inositol (D-P4), can be conveniently prepared from the chiral natural products 1D-pinitol or 1D-chiro-inositol. On treatment of toluene solutions of RuCl2(PPh3)3 with two mole equivalents of the ligands D-PY (Y = 1-4) the complexes RuCl2(D-P1)2 (1), RuCl2(D-P2)2 (4), RuCl2(D-P3)2 (5), or RuCl2(D-P4)2 (6), respectively, are formed. Similarly, treatment of OsCl2(PPh3)3 with D-P1 gives OsCl2(D-P1)2 (7). The single crystal X-ray structure determination of 1 reveals that each D-P1 ligand coordinates to ruthenium through phosphorus and the oxygen atom of the methoxyl group. Treatment of 1 with excess LiBr or LiI results in metathesis of the chloride ligands and RuBr2(D-P1)2 (2) or RuI2(D-P1)2 (3), respectively, are formed. Exposure of a solution of 1 to carbon monoxide results in the very rapid formation of RuCl2(CO)2(D-P1)2 (8), thereby demonstrating the ease with which the oxygen donors are displaced from the metal and hence the hemilabile nature of the two bidentate D-P1 ligands in 1. Preliminary studies indicate that 1-7 act as catalysts for the asymmetric hydrogenation reactions of acetophenone and 3-Quinuclidinone to give the corresponding alcohols in generally high conversions but low enantiomeric excesses.
Structural basis for high substrate-binding affinity and enantioselectivity of 3-quinuclidinone reductase AtQR.[Pubmed:24642255]
Biochem Biophys Res Commun. 2014 Apr 18;446(4):911-5.
(R)-3-Quinuclidinol, a useful compound for the synthesis of various pharmaceuticals, can be enantioselectively produced from 3-Quinuclidinone by 3-Quinuclidinone reductase. Recently, a novel NADH-dependent 3-quinuclidionone reductase (AtQR) was isolated from Agrobacterium tumefaciens, and showed much higher substrate-binding affinity (>100 fold) than the reported 3-quinuclidionone reductase (RrQR) from Rhodotorula rubra. Here, we report the crystal structure of AtQR at 1.72 A. Three NADH-bound protomers and one NADH-free protomer form a tetrameric structure in an asymmetric unit of crystals. NADH not only acts as a proton donor, but also contributes to the stability of the alpha7 helix. This helix is a unique and functionally significant part of AtQR and is related to form a deep catalytic cavity. AtQR has all three catalytic residues of the short-chain dehydrogenases/reductases family and the hydrophobic wall for the enantioselective reduction of 3-Quinuclidinone as well as RrQR. An additional residue on the alpha7 helix, Glu197, exists near the active site of AtQR. This acidic residue is considered to form a direct interaction with the amine part of 3-Quinuclidinone, which contributes to substrate orientation and enhancement of substrate-binding affinity. Mutational analyses also support that Glu197 is an indispensable residue for the activity.
Structural basis of stereospecific reduction by quinuclidinone reductase.[Pubmed:24507746]
AMB Express. 2014 Feb 7;4(1):6.
Chiral molecule (R)-3-quinuclidinol, a valuable compound for the production of various pharmaceuticals, is efficiently synthesized from 3-Quinuclidinone by using NADPH-dependent 3-Quinuclidinone reductase (RrQR) from Rhodotorula rubra. Here, we report the crystal structure of RrQR and the structure-based mutational analysis. The enzyme forms a tetramer, in which the core of each protomer exhibits the alpha/beta Rossmann fold and contains one molecule of NADPH, whereas the characteristic substructures of a small lobe and a variable loop are localized around the substrate-binding site. Modeling and mutation analyses of the catalytic site indicated that the hydrophobicity of two residues, I167 and F212, determines the substrate-binding orientation as well as the substrate-binding affinity. Our results revealed that the characteristic substrate-binding pocket composed of hydrophobic amino acid residues ensures substrate docking for the stereospecific reaction of RrQR in spite of its loose interaction with the substrate.
Highly efficient synthesis of (R)-3-quinuclidinol in a space-time yield of 916 g L(-1) d(-1) using a new bacterial reductase ArQR.[Pubmed:24050153]
Org Lett. 2013 Oct 4;15(19):4917-9.
A new keto reductase (ArQR), identified from Agrobacterium radiobacter ECU2556, can efficiently reduce 3-Quinuclidinone in excellent enantioselectivity and high space-time yield for the synthesis of (R)-3-quinuclidinol, a chiral building block of many antimuscarinic agents. This is the first time that a high yield of (R)-3-quinuclidinol up to 916 g L(-1) d(-1) using a bioreduction approach is reported.
Functional characterization of a novel tropinone reductase-like gene in Dendrobium nobile Lindl.[Pubmed:23566874]
J Plant Physiol. 2013 Jul 1;170(10):958-64.
Dendrobium nobile, a herbal medicine plant, contains many important alkaloids and other secondary metabolites with pharmacological and clinical effects. However, the biosynthetic pathway of these secondary metabolites is largely unknown. In present study, a cDNA sequence (DnTR2) that encodes a peptide with high similarity to known tropinone reductase (TR) was cloned from D. nobile Lindl. Sequence comparison and phylogenetic analysis showed that DnTR2 was evolutionarily distant from those well-characterized subgroups of TRs. qRT-PCR revealed that DnTR2 was expressed constitutively in all three vegetative organs (leaves, stems and roots) and was regulated by methyl jasmonate (MeJA), salicylic acid (SA) and nitrogen oxide (NO). Catalytic activity analysis using recombinant protein found that DnTR2 was not able to reduce tropinone, but reduced the two structural analogs of tropinone, 3-Quinuclidinone hydrochloride and 4-methylcyclohexanone. Structural modeling and comparison suggested that the substrate specificity of TRs may not be determined by their phylogenetic relationships but by the amino acids that compose the substrate binding pocket. To verify this hypothesis, a site-directed mutagenesis was performed and it successfully restored the DnTR2 with tropinone reduction activity. Our results also showed that the substrate specificity of TRs was determined by a few residues that compose the substrate binding pocket which may have an important role for directed selecting of TRs with designated substrate specificities.
Gene cloning and characterization of two NADH-dependent 3-quinuclidinone reductases from Microbacterium luteolum JCM 9174.[Pubmed:23263947]
Appl Environ Microbiol. 2013 Feb;79(4):1378-84.
We used the resting-cell reaction to screen approximately 200 microorganisms for biocatalysts which reduce 3-Quinuclidinone to optically pure (R)-(-)-3-quinuclidinol. Microbacterium luteolum JCM 9174 was selected as the most suitable organism. The genes encoding the protein products that reduced 3-Quinuclidinone were isolated from M. luteolum JCM 9174. The bacC gene, which consists of 768 nucleotides corresponding to 255 amino acid residues and is a constituent of the bacilysin synthetic gene cluster, was amplified by PCR based on homology to known genes. The qnr gene consisted of 759 nucleotides corresponding to 252 amino acid residues. Both enzymes belong to the short-chain alcohol dehydrogenase/reductase (SDR) family. The genes were expressed in Escherichia coli as proteins which were His tagged at the N terminus, and the recombinant enzymes were purified and characterized. Both enzymes showed narrow substrate specificity and high stereoselectivity for the reduction of 3-Quinuclidinone to (R)-(-)-3-quinuclidinol.
Production of (R)-3-quinuclidinol by E. coli biocatalysts possessing NADH-dependent 3-quinuclidinone reductase (QNR or bacC) from Microbacterium luteolum and Leifsonia alcohol dehydrogenase (LSADH).[Pubmed:23202966]
Int J Mol Sci. 2012 Oct 19;13(10):13542-53.
We found two NADH-dependent reductases (QNR and bacC) in Microbacterium luteolum JCM 9174 (M. luteolum JCM 9174) that can reduce 3-Quinuclidinone to optically pure (R)-(-)-3-quinuclidinol. Alcohol dehydrogenase from Leifsonia sp. (LSADH) was combined with these reductases to regenerate NAD+ to NADH in situ in the presence of 2-propanol as a hydrogen donor. The reductase and LSADH genes were efficiently expressed in E. coli cells. A number of constructed E. coli biocatalysts (intact or immobilized) were applied to the resting cell reaction and optimized. Under the optimized conditions, (R)-(-)-3-quinuclidinol was synthesized from 3-Quinuclidinone (15% w/v, 939 mM) giving a conversion yield of 100% for immobilized QNR. The optical purity of the (R)-(-)-3-quinuclidinol produced by the enzymatic reactions was >99.9%. Thus, E. coli biocatalysis should be useful for the practical production of the pharmaceutically important intermediate, (R)-(-)-3-quinuclidinol.
Molecular cloning and characterization of a tropinone reductase from Dendrobium nobile Lindl.[Pubmed:23104472]
Mol Biol Rep. 2013 Feb;40(2):1145-54.
A cDNA sequence that encodes a peptide with similarity to known tropinone reductases (TR) was cloned from Dendrobium nobile Lindl. The full coding region of the gene (DnTR1) is 804 bp in length which encodes a putative peptide consisting of 268 amino acids. Phylogenetic analysis showed that DnTR1 was a novel member of the TR family and evolutionarily distant from those well-characterized subgroups of TRs, suggesting that DnTR1 may have distinct characteristics. Structural modeling found that DnTR1 had a similar electrostatic environment at the inner molecular surface of the substrate binding pocket with TRI encoded by Datura stramonium (DsTRI). Catalytic activity assay with recombinant protein demonstrated that DnTR1 was able to reduce tropinone, 3-Quinuclidinone hydrochloride, and 4-methylcyclohexanone using NADPH as coenzyme. Gene expression profiling by qRT-PCR revealed that the DnTR1 transcript was expressed in all three vegetative organs (leaves, stems and roots) of D. nobile with the highest expression level in roots. The expression of DnTR1 mRNA was enhanced 9.5 times (P < 0.01) by treatment of methyl jasmonate at 24 h, but not affected by salicylic acid and sodium nitroprusside treatments, indicating that DnTR1 regulation may be involved in a jasmonate-dependent pathway.
Expression, purification, crystallization and X-ray analysis of 3-quinuclidinone reductase from Agrobacterium tumefaciens.[Pubmed:23027756]
Acta Crystallogr Sect F Struct Biol Cryst Commun. 2012 Oct 1;68(Pt 10):1237-9.
(R)-3-Quinuclidinol is a useful chiral building block for the synthesis of various pharmaceuticals and can be produced from 3-Quinuclidinone by asymmetric reduction. A novel 3-Quinuclidinone reductase from Agrobacterium tumefaciens (AtQR) catalyzes the stereospecific reduction of 3-Quinuclidinone to (R)-3-quinuclidinol with NADH as a cofactor. Recombinant AtQR was overexpressed in Escherichia coli, purified and crystallized with NADH using the sitting-drop vapour-diffusion method at 293 K. Crystals were obtained using a reservoir solution containing PEG 3350 as a precipitant. X-ray diffraction data were collected to 1.72 A resolution on beamline BL-5A at the Photon Factory. The crystal belonged to space group P2(1), with unit-cell parameters a = 62.0, b = 126.4, c = 62.0 A, beta = 110.5 degrees , and was suggested to contain four molecules in the asymmetric unit (V(M) = 2.08 A(3) Da(-1)).
Effect of buffer general acid-base catalysis on the stereoselectivity of ester and thioester H/D exchange in D2O.[Pubmed:21384891]
J Am Chem Soc. 2011 Apr 6;133(13):5124-8.
As part of a comprehensive investigation on the stereochemistry of base-catalyzed 1,2-elimination and H/D exchange reactions of carbonyl compounds, we have found that the stereoselectivity of H/D exchange of 3-hydroxybutyryl N-acetylcysteamine (3) in D(2)O is strongly influenced by the presence of buffers. This buffer effect is also operative with a simple acyclic ester, ethyl 3-methoxybutanoate (7). Buffers whose general-acid components are cyclic tertiary ammonium ions are particularly effective in changing the stereoselectivity. (2)H NMR analysis showed that without buffer, H/D exchange of 3 produces 81-82% of the 2R*, 3R* diastereomer of 2-deuterio 3 (the anti product). In the presence of 0.33 M 3-Quinuclidinone buffer, only 44% of the 2R*, 3R* diastereomer was formed. With ester 7, the stereoselectivity went from 93-94% in DO(-)/D(2)O to 60% in the presence of buffer. Phosphate buffer, as well as others, also showed substantial effects. The results are put into the context of what is known about the mechanism of H/D exchange of esters and thioesters, and the relevance of the buffer effect on the mechanism of the enoyl-CoA hydratase reaction is discussed. It is likely that hydrogen bonding in the enolate-buffer acid encounter complex is an important stereochemical determinant in producing a greater amount of the 2R*, 3S* diastereomer (the syn product). Studies that involve the protonation of enolate anions in D(2)O need to include the buffer general acid in any understanding of the stereoselectivity.
Determination of the pKa of cyclobutanone: Bronsted correlation of the general base-catalyzed enolization in aqueous solution and the effect of ring strain.[Pubmed:21162595]
J Org Chem. 2011 Jan 21;76(2):380-90.
The induction of strain in carbocycles, thereby increasing the amount of s-character in the C-H bonds and the acidity of these protons, has been probed with regard to its effect on the rate constants for the enolization of cyclobutanone. The second-order rate constants for the general base-catalyzed enolization of cyclobutanone have been determined for a series of 3-substituted quinuclidine buffers in D(2)O at 25 degrees C, I = 1.0 M (KCl). The rate constants for enolization were determined by following the extent of deuterium incorporation (up to approximately 30% of the first alpha-proton) into the alpha-position, as a function of time. The observed pseudo-first-order rate constants correlated to the [basic form] of the buffer and yielded the second-order rate constants for the general base-catalyzed enolization of cyclobutanone for four tertiary amine buffers. A Bronsted beta-value of 0.59 was determined from the second-order rate constants determined. Comparison of the results for cyclobutanone to those previously reported for acetone and a 1-phenylacetone derivative, under similar conditions, indicated that the ring strain of the carbocycle appeared to have only a small effect on the general base-catalyzed rate constants for enolization. The similarity of the rate constants for the general base-catalyzed enolization of cyclobutanone to those determined for acetone allowed for an estimation of the limits of the rate constant for protonation of the enolate intermediate of cyclobutanone by the conjugate acid of 3-Quinuclidinone (k(BH) = 5 x 10(8) - 2 x 10(9) M(-1) s(-1)). Combining the rate constants for deprotonation of cyclobutanone (k(B)) and protonation of the enolate of cyclobutanone (k(BH)) by 3-Quinuclidinone and its conjugate acid, the pK(a) of the alpha-protons of cyclobutanone has been estimated to be pK(a) = 19.7-20.2.
Asymmetric hydrogenation of bicyclic ketones catalyzed by BINAP/IPHAN-Ru(II) complex.[Pubmed:20670005]
Org Lett. 2010 Aug 6;12(15):3380-3.
Hydrogenation of 3-Quinuclidinone and bicyclo[2.2.2]octan-2-one with a combined catalyst system of RuCl(2)[(S)-binap][(R)-iphan] and t-C(4)H(9)OK in 2-propanol afforded the chiral alcohols in 97-98% ee. 2-Diphenylmethyl-3-Quinuclidinone was hydrogenated with the same catalyst to the cis alcohol with perfect diastereo- and enantioselectivity. The reaction of unsymmetrical ketones with a bicyclo[2.2.1] or -[2.2.2] skeleton gave the corresponding alcohols with high stereoselectivity.